What are viruses?
Viruses must infect a host to multiply.
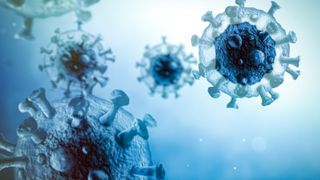
Viruses are microscopic infectious agents that contain genetic material, either DNA or RNA, and must invade a host in order to multiply.
Predominantly, viruses are known for causing disease, as they've triggered widespread outbreaks of illness and death throughout human history. Recent examples of virus-driven outbreaks include the 2014 Ebola outbreak in West Africa, the 2009 swine flu pandemic and the COVID-19 pandemic, which was caused by a coronavirus first identified in late 2019.
While such viruses certainly are wily foes for scientists and medical professionals, others of their ilk have been instrumental as research tools that have helped further the understanding of basic cellular processes, such as the mechanics of protein synthesis, and of viruses themselves.
Related: 20 of the worst epidemics and pandemics in history
How were viruses discovered?
Toward the end of the 19th century, the notion that microorganisms, especially bacteria, could cause disease was well established, according to the Smithsonian Magazine. However, researchers looking into a troubling disease in tobacco plants — named tobacco mosaic disease — were somewhat stumped as to its cause, according to "Discoveries in Plant Biology" (World Publishing Co., 1998).
In an 1886 research paper titled "Concerning the Mosaic Disease of Tobacco," Adolf Mayer, a German chemist and agricultural researcher, published the results of his extensive experiments on tobacco plants affected by the disease, which caused the plants' leaves to break out in dark green, yellow and gray splotches. Mayer found that, when he crushed up infected tobacco leaves and injected the resulting juice into the veins of healthy leaves, the once-healthy leaves developed the speckling and discoloration characteristic of the diseased plants. Mayer correctly surmised that whatever was causing tobacco mosaic disease was in the leafy juice.
However, more concrete results eluded him. Based on the previous work of German physician Robert Koch, who discovered the bacteria that causes tuberculosis, Mayer thought he should be able to isolate and grow the pathogen behind tobacco mosaic disease in lab dishes. However, he was unable to isolate the disease-causing agent or identify it under a microscope. Nor could he recreate the disease by injecting healthy plants with a range of known bacteria, according to Smithsonian Magazine.
In 1892, a Russian student named Dmitri Ivanovsky (sometimes written as Ivanowski) essentially repeated Mayer’s juicing experiments but with a bit of a twist.
According to a 1972 article published in the journal Bacteriological Reviews, Ivanovsky passed the juice from infected leaves through a Chamberland filter, which is fine enough to capture bacteria and other known microorganisms. Despite the sieving, the liquid filtrate remained infectious, suggesting a new piece to the puzzle: Whatever was causing the disease was small enough to pass through the filter.
However, Ivanovsky also concluded that the cause of tobacco mosaic disease was bacterial, suggesting the filtrate "contained either bacteria or a soluble toxin." It wasn’t until 1898 that the existence of viruses was acknowledged. Dutch scientist Martinus Beijerinck, while confirming Ivanovsky’s results, suggested that the cause of tobacco mosaic disease was not bacterial but a "contagium vivum fluidum," or a contagious living fluid, according to "Discoveries in Plant Biology." He referred to this fluid as "virus," for short, derived from the Latin word for liquid poison, according to Smithsonian Magazine.
The experiments of Mayer, Ivanovsky, Beijerinck and others that followed only pointed to the existence of viruses; it would take a few more decades before anyone actually saw a virus.
In 1935, chemist Wendell M. Stanley crystallized a sample of the tobacco mosaic virus such that the pathogen could be seen on X-ray, according to Smithsonian Magazine. However, it wasn't until 1939 that the first clear snapshots of the unaltered virus could be captured. This feat was made possible by the invention of the electron microscope, an instrument that uses beams of negatively charged particles to produce images of extremely small objects, according to a 2009 article published in the journal Clinical Microbiology Reviews.
How big are viruses?
How much smaller are most viruses in comparison to bacteria? Quite a bit.
With a diameter of 220 nanometers, the measles virus is about eight times smaller than Escherichia coli bacteria; for reference, one nanometer is equal to 0.000000039 inches. At 45 nm, the hepatitis virus is about 40 times smaller than E. coli. For a sense of how small this is, David R. Wessner, a professor of biology at Davidson College, provides an analogy in a 2010 article published in the journal Nature Education: The poliovirus, 30 nm across, is about 10,000 times smaller than a grain of salt.
Although most viruses are considerably smaller than bacteria, in the 1990s, scientists uncovered humongous viruses that rivaled bacteria in size, according to the Nature Education report.
In 1992, scientists discovered bacteria-like structures within some amoebas from a water-cooling tower. A later analysis of the bacteria-like entities, published in 2003, revealed that these strange structures weren't bacteria at all, but were actually giant viruses. The researchers named the huge virus Acanthamoeba polyphaga mimivirus (APMV).
After the discovery of APMV, which measures 750 nm in diameter, researchers found more large viruses, including a second strain of APMV dubbed "mamavirus," according to the Nature Education report. To date, there are four known giant virus families: Mollivirus, Megavirus, Pithovirus and Pandoravirus. Giant viruses have been found in exotic environments, from melting permafrost in Siberia to the depths of the Antarctic ocean, and have mostly been found to infect amoebas and phytoplankton, although lab studies hint that they could also infect animal cells. Research suggests that giant viruses may invent genes and proteins found nowhere else on Earth, and that they spew out these genes through a star-shaped gate on their surfaces.
Are viruses alive?
Viruses teeter on the boundaries of what is considered life. On one hand, viruses contain either DNA or RNA, the nucleic acids found in all living organisms. On the other hand, viruses lack the capacity to independently read and act upon the information contained within those nucleic acids; for this reason, viruses aren't considered "alive."
Related: Are viruses alive?
How are viruses structured?
When a virus is completely assembled and capable of infection, it is known as a virion. According to "Medical Microbiology" (University of Texas Medical Branch at Galveston, 1996), simple virions contain an inner nucleic acid core surrounded by an outer casing of proteins known as the capsid. Capsids protect viral nucleic acids from being chewed up and destroyed by special enzymes in the host cell called nucleases.
Some viruses have a second protective layer known as the envelope. This layer is usually derived from the cell membrane of a host; little stolen bits that are modified and repurposed for the virus to use.
The DNA or RNA found in the inner core constitutes the virus's genome, or the sum total of its genetic information. Viral genomes are generally small in size, coding only for essential proteins such as capsid proteins, enzymes and proteins necessary for replication within a host cell.
However, giant virus genomes can be much, much larger than those of typical viruses. For example, APMV carries approximately 1.2 million base pairs in its genome, where each "base pair" is one rung in the twisted ladder of DNA. The poliovirus genome, by comparison, is only 7,500 nucleotides long and the smallpox virus genome is 200,000 nucleotides long, according to the Nature Education report.
How do viruses work?
A virus requires a host cell to replicate, or make more copies of itself, said Jaquelin Dudley, a professor of molecular biosciences at the University of Texas at Austin. "The virus cannot reproduce itself outside the host because it lacks the complicated machinery that a [host] cell possesses," she told Live Science. The host cell's cellular machinery allows viruses to produce RNA from their DNA (a process called transcription) and to build proteins based on the instructions encoded in their RNA (a process called translation).
Therefore, the primary role of a virus is to "deliver its DNA or RNA genome into the host cell so that the genome can be expressed (transcribed and translated) by the host cell," according to "Medical Microbiology."
First, viruses break into the host cell, which may be part of a larger organism, in the case of animals and humans. Respiratory passages and open wounds can act as gateways for viruses into the body. And sometimes, insects provide the mode of entry; certain viruses will hitch a ride in an insect’s saliva and enter the host’s body after the insect bites. According to "Molecular Biology of the Cell" (Garland Science, 2002), such viruses can replicate inside both insect and host cells, ensuring a smooth transition from one to the other. Examples of such pathogens include the viruses that cause yellow fever and dengue fever.
Once inside an organism, viruses will then attach themselves to the surface of host cells. They do so by recognizing and binding to cell surface receptors, or proteins that stick off the cell surface; proteins on the viral surface fit onto these receptors like interlocking puzzle pieces. Many different viruses can bind to the same receptor and a single virus can bind different cell surface receptors. While viruses use them to their advantage, cell surface receptors are actually designed to serve the cell.
After a virus binds to the surface of the host cell, it can start to move across the outer covering or membrane of the host cell. There are many different modes of entry. HIV, a virus with an envelope, fuses with the host cell membrane and is pushed through. Another enveloped virus, the influenza virus, is engulfed by the cell. Some non-enveloped viruses, such as the poliovirus, create a porous channel of entry and burrow through the membrane, according to "Molecular Biology of the Cell."
Once inside the host cell, viruses disrupt or hijack various parts of the cellular machinery inside. Viral genomes direct host cells to produce viral proteins, and this often halts the synthesis of any RNA and proteins that the host cell can use for its own purposes.
Originally published on Live Science.
This article was updated on May 13, 2022 by Live Science contributor Nicoletta Lanese.
New discoveries
- Scientists recently identified more than 5,000 new RNA viruses in the world's oceans.
- The Epstein-Barr virus, which causes "mono," may be an underlying cause of multiple sclerosis, an autoimmune disease.
- A newfound variant of HIV has been uncovered in the Netherlands and appears to cause faster disease progression compared with other versions of the virus.
- Doctors treated a patient's chronic wound with bacteriophages, or viruses that infect bacteria.
Additional resources
- Read about the multitude of viruses that exist inside and all around us in Carl Zimmer's book "A Planet of Viruses."
- Find out what would happen if all the viruses in the world vanished in this SciShow video.
- Read "Spillover: Animal Infections and the Next Human Pandemic" by David Quammen to learn about deadly viral diseases that have jumped from animals to humans.
Bibliography
Alberts, B., Johnson, A., Lewis, J., Raff, M., Roberts, K., & Walter, P. (2002). Cell Biology of Infection. In Molecular Biology of the Cell (4th ed.). essay, Garland Science.
Gelderblom, H. R. (1996). Structure and Classification of Viruses. In Medical Microbiology (4th ed.). essay, University of Texas Medical Branch at Galveston.
Goldsmith, C. S., & Miller, S. E. (2009). Modern Uses of Electron Microscopy for Detection of Viruses. Clinical Microbiology Reviews, 22(4), 552–563. https://doi.org/10.1128/cmr.00027-09
Lechevalier, H. (1972). Dmitri Iosifovich Ivanovski (1864-1920). Bacteriological Reviews, 36(2), 135–145. https://doi.org/10.1128/br.36.2.135-145.1972
Machemer, T. (2020, March 24). How a Few Sick Tobacco Plants Led Scientists to Unravel the Truth About Viruses. Smithsonian Magazine. Retrieved May 12, 2022, from https://www.smithsonianmag.com/science-nature/what-are-viruses-history-tobacco-mosaic-disease-180974480/
Wessner, D. R. (2010). Discovery of the Giant Mimivirus. Nature Education, 3(9). https://www.nature.com/scitable/topicpage/discovery-of-the-giant-mimivirus-14402410/
Zaitlin, M. (1998). The Discovery of the Causal Agent of the Tobacco Mosaic Disease. In Discoveries in Plant Biology (pp. 105–110). essay, World Publishing Co.
Sign up for the Live Science daily newsletter now
Get the world’s most fascinating discoveries delivered straight to your inbox.
Aparna Vidyasagar is a freelance science journalist who specializes in health and life sciences. Aparna has written for a number of publications, including New Scientist, Science, PBS SoCal, Mental Floss, and several others. Aparna has a doctorate in Cellular and Molecular Pathology from the University of Wisconsin-Madison, and also received a master’s degree and bachelor’s degree from the same university.
- Nicoletta LaneseChannel Editor, Health