CRISPR 'will provide cures for genetic diseases that were incurable before,' says renowned biochemist Virginijus Šikšnys
Live Science spoke with biochemist Virginijus Šikšnys, whose work helped establish CRISPR as a gene-editing system.
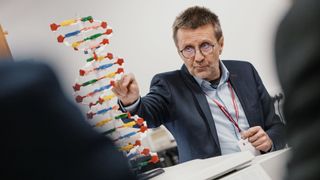
Scientists introduced CRISPR to the world as a gene-editing tool in summer 2012, when landmark papers from two independent groups demonstrated how the system could be wielded to make cuts in DNA. Now, less than 12 years later, we're seeing CRISPR put to use in groundbreaking medical treatments.
Virginijus Šikšnys was a senior author of one of those paradigm-shifting papers.
"It's really rewarding to see how fast the fundamental discoveries that were made in the lab actually are translated into the clinics," said Šikšnys, who is chief scientist and head of the Department of Protein-DNA Interactions at the Vilnius University Institute of Biotechnology in Lithuania.
Prior to those seminal papers, other researchers had begun unraveling how CRISPR works inside microbes. Although best known as a gene-editing tool, CRISPR was first found in bacteria, and scientists realized that it acts as a kind of immune system — a defense against viruses. In this immune system, the bacterium has a memory bank full of virus' genetic material. The bacterium will stash away this material after being attacked by a virus so it can guard against future invasions.
This memory bank is paired with tiny, molecular scissors called Cas proteins that snip through DNA, and a molecule that guides the scissors to their target. In bacteria, that target is a viral invader. But Šikšnys and his colleagues demonstrated that scientists could co-opt these scissors for their own purposes, targeting any DNA they want to edit. They specifically showed this with the protein Cas9.
Alongside Jennifer Doudna and Emmanuelle Charpentier — authors of the other groundbreaking CRISPR paper published in 2012 — Šikšnys was awarded the 2018 Kavli Prize in Nanoscience for the invention of CRISPR-Cas9, "a precise nanotool for editing DNA." Nowadays, he and his team are investigating the diversity of CRISPR systems that exist in nature to see which others might be useful for engineering genomes.
Live Science spoke with Šikšnys about what it's been like to see CRISPR enter clinical use and how he thinks the system might be applied and improved upon in the future.
Sign up for the Live Science daily newsletter now
Get the world’s most fascinating discoveries delivered straight to your inbox.
Editor's Note: This interview has been condensed and edited for clarity.
Related: Gene therapy: What is it and how does it work?
Nicoletta Lanese: Could you describe when you first began working with CRISPR-Cas? And could you give a sense of when you clued into the idea that this could be "a big deal" — a big-ticket technology that kind of shifts gene-editing as we know it?
Virginijus Šikšnys: We jumped into the CRISPR field, I would say, from the very beginning. It happened probably in 2007 when a paper appeared in Science, describing for the first time the possible function of the CRISPR-Cas system as an antiviral defense system in bacteria. And we decided to look, actually, at how this system functioned. This is how we started our CRISPR journey.
Of course, in the very beginning, we were very much interested in very basic biological questions. ... It took us a while to understand the mechanisms behind the CRISPR-Cas systems. …
In [our 2012] paper, we showed that we can reprogram Cas9 protein and address it to any sequence in the genome. This was probably the moment where we understood that, indeed, this is a kind of really versatile system that could be employed for genome editing in different model organisms. And this is how, then, this kind of gene-editing field started.
NL: Did you envision right away that this might be applied in the treatment of genetic disorders? Did you see that as a possibility, even early on?
VS: If I recall, what we put in our paper at that time — we said that these CRISPR-Cas systems, or Cas9 protein programmed by CRISPR RNA, could be used for precise "DNA surgery."
It means that, actually, you can direct Cas9 to any sequence in the genome, including the sequences where [there are] mutations that cause genetic disease.
NL: Having seen, within the last year, the first CRISPR-based therapies come to market — I'm wondering how it feels to have seen the progression of the field from that basic research to now seeing it applied at that level?
VS: Indeed, looking backwards, it's really amazing to see that Cas9 made it into the clinics, nearly, in 10 years. I think it's a really great achievement, and I'm sure that more therapeutic applications will follow in the near future and will provide cure[s] for genetic diseases that were incurable before.
And if you look at the list of clinical trials that are currently ongoing, where the Cas9 genome-editing tool is employed to treat different genetic diseases — the list is really very impressive. And it's really rewarding to see how fast the fundamental discoveries that were made in the lab actually are translated into the clinics.
NL: Having seen the graduation into the clinic now, how do you anticipate the gene-editing systems borne of CRISPR-Cas might be refined in the future?
VS: Indeed, the CRISPR-Cas9 technology is a great tool that is rapidly advancing into the clinics. But still, there are several challenges that need to be overcome, and there are, of course, avenues for improvement of this tool. …
Recently, it made headlines that that CRISPR tool was used for treatment of SCD disease [sickle-cell disease]. In fact, [it] showed that this is really a tool that could be used in the clinics for the treatment of the patients.
But of course, this treatment has several limitations, because, in this case, the treatment is occuring ex vivo. It means that the cells that need to be treated are removed from the patient's body, then Cas9 tool is applied to correct the mutation — or actually, trigger production of fetal hemoglobin. And then these engineered cells, they have to be delivered back to the patient's body. And of course, this is kind of a challenging and time-consuming procedure.
So of course, it would be great if the CRISPR treatment could be done directly in [the] human body — we call it in vivo. But actually, to do that, you have to overcome several challenges: First, you have to deliver this CRISPR tool into specific tissues or organs in human body. And of course, there are many ways to deliver CRISPR tools, but after COVID, mRNA vaccines were approved as a therapeutic modality for treatment [prevention] of COVID. And currently, mRNA coupled with lipid nanoparticles became one of the key modalities that could deliver Cas9 into different cells and tissues in a human body.
[Other] delivery systems are also [being] explored, including virus-like particles and adeno-associated viruses. So AAVs are also used as delivery tools and they are approved as safe delivery tools into human body — but, for example, in the case of AAV, there is a packaging cargo limitation and you need to find smaller gene editing tools that could be packaged into a single AAV particle.
In my lab, in fact, we are looking at the avenues — how do you improve the existing tools or, actually, find new tools? To find new tools, we look at the diversity of CRISPR-Cas systems. These CRISPR-Cas systems [in nature] are very, very diverse, and we aim to understand this diversity of CRISPR-Cas systems from a fundamental perspective. And also, we hope that, looking at this diversity, we'll be able to find new tools for genome editing applications.
Related: Meet 'Fanzor,' the 1st CRISPR-like system found in complex life
NL: Could you paint a picture of what it looks like to dig into the diversity of these systems?
VS: In my lab, we're using a combined bioinformatics-based, biochemical approach. So we try to identify putative new CRISPR-Cas systems bioinformatically, and then, we try to characterize them biochemically using the tools available in the wet lab. …
First, we look at [microbial DNA] sequences that are present in really huge databases — you can just try to find new CRISPR systems there. Then, we try to express them in different bacteria, isolate them, characterize, and then move them to human cells to see whether they can be applied as new genome modification tools.
NL: We touched on the sickle cell treatments that have just been approved — I'm wondering if you, like others, anticipated that sickle cell would be one of the first diseases to get a CRISPR treatment? And what diseases do you see as the next frontier?
VS: I would say that it was clear from the very beginning that genetic diseases that are caused by a single mutation, like sickle-cell disease, will be the first target. It looked like low-hanging fruit, because you have to correct just a single mutation in the genome. And of course, I think part of the credit for this Cas9-based treatment of sickle-cell disease should also go toward the people who were studying sickle-cell disease for decades. They were providing us with understanding of the mechanisms of the disease that were harnessed into the treatment.
The other reason why SCD was a clear target was because, as I mentioned before, you can do the treatment [ex] vivo. You can remove cells that contain [the] mutation, actually engineer them in the lab, and put the cells back into human body. So that makes manipulations easier.
But of course, when you think about the next step — treatment of genetic diseases that are caused by multiple mutations, like cancer, for example, is still a challenge. But of course, scientists are trying to develop approaches how to tackle such complex genetic disease. And, for example, T-cell-based therapies are already in the clinics, and CRISPR [systems] are used there to facilitate engineering of these T cells … that could be used to treat cancers like lymphomas and solid tumors.
And of course, the CRISPR treatments in the human body, as I discussed before, is the next big step.
The beauty of CRISPR-Cas9 technology is that it's a kind of versatile, or universal technology, because you can use this tool to engineer any living organism.
Virginijus Šikšnys, Vilnius University
NL: This is somewhat tangential, but we've covered the idea of developing CRISPR as an antibacterial, as a kind of alternate antibiotic — do you see that as a fruitful research area?
VS: The beauty of CRISPR-Cas9 technology is that it's a kind of versatile, or universal technology, because you can use this tool to engineer any living organism. You are just trying to engineer DNA, and DNA is the blueprint of every living organism. So instead of doing gene-editing in human cells, you can also think about doing editing of bacterial population — let's say, that are present in [the] human gut. And these bacterial populations could be engineered. …
And as you mentioned, Cas9, CRISPR technologies could be used also as antiviral agents. Currently, the problem with antibiotics is pretty clear — we are probably losing our battle against bacteria using antibiotics. Novel antibiotics are always required and it's really difficult to find them, and challenging and costly. So therefore, alternative technologies like viral therapies or CRISPR antibacterial systems are developed.
NL: Obviously CRISPR has so much potential, especially in the realm of treating genetic disease — I think people also have a lot of questions about the ethics of applying CRISPR in different contexts. Could you speak on that?
VS: I think it's a very important question, and of course, CRISPR is a very important technology, and you can use CRISPR to do many things. But of course, you should keep in mind what you are doing and you need to be in touch and in conversation with society — Are these things acceptable? Or, what are the societal views on these technologies that scientists are developing in the lab? And I think it's very important to communicate with people and explain, actually, what are these technologies, what they can achieve, and then what can be downsides, also, of these technologies.
We already heard about these stories that CRISPR was used several years ago in China for engineering human embryos — so that's a line that scientists actually agree not to cross, because this could be a really dangerous thing.
Ever wonder why some people build muscle more easily than others or why freckles come out in the sun? Send us your questions about how the human body works to community@livescience.com with the subject line "Health Desk Q," and you may see your question answered on the website!
Nicoletta Lanese is the health channel editor at Live Science and was previously a news editor and staff writer at the site. She holds a graduate certificate in science communication from UC Santa Cruz and degrees in neuroscience and dance from the University of Florida. Her work has appeared in The Scientist, Science News, the Mercury News, Mongabay and Stanford Medicine Magazine, among other outlets. Based in NYC, she also remains heavily involved in dance and performs in local choreographers' work.