New discovery could help take down drug-resistant bacteria
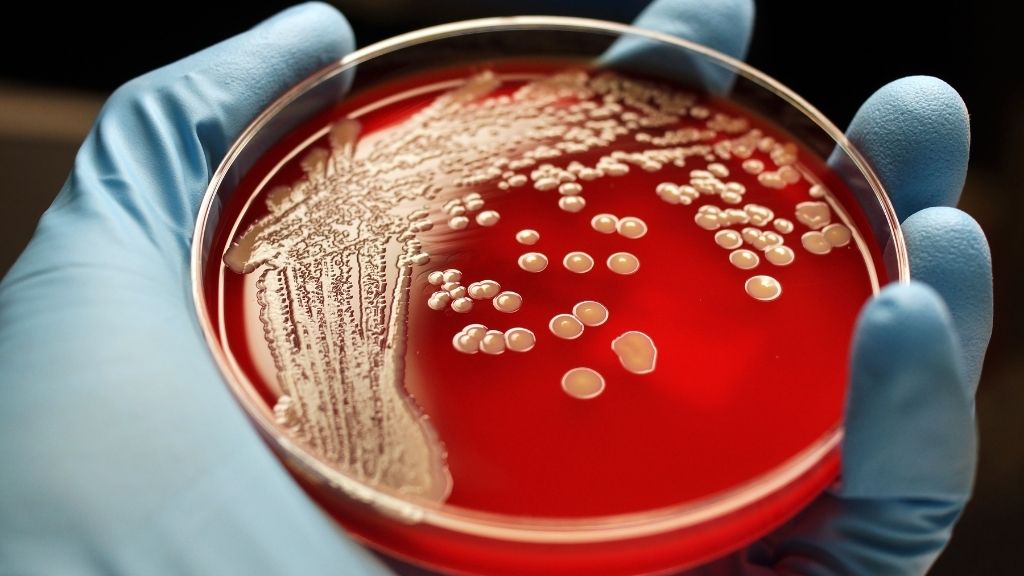
Scientists have found a new way to kill antibiotic-resistant bacteria. The new approach disarms their natural defense mechanism, making existing antibiotics more lethal.
The study, conducted in lab dishes and mice, offers a promising strategy for taking down so-called superbugs without needing to make brand-new antibiotics.
"You want to make the already existing antibiotics with good safety profiles more potent," and with the help of a few newfound chemicals, the research team did just that, said senior author Evgeny Nudler, a professor of biochemistry at the New York University Grossman School of Medicine and an investigator with the Howard Hughes Medical Institute.
In the new study, published Thursday (June 10) in the journal Science, the team took aim at Staphylococcus aureus and Pseudomonas aeruginosa, two bacteria that show pervasive resistance to multiple drugs and rank among the leading causes of hospital-acquired infections. These bacteria rely on an enzyme called cystathionine gamma-lyase (CSE) to counter the toxic effects of bactericidal antibiotics, drugs that kill bacteria rather than just slowing their growth.
Related: 6 superbugs to watch out for
Specifically, the enzyme produces hydrogen sulfide, a compound that shields bacteria from oxidative stress, or an accumulation of free radicals. So the team sifted through more than 3 million small molecules to find chemicals that would block CSE without interacting with mammalian cells, and they found three strong candidates.
In lab dishes, the newfound molecules made bactericidal antibiotics two- to 15-fold more potent against the microbes, depending on the antibiotic being used and the bacterial strain being targeted. One of the small molecules also improved the survival of antibiotic-treated mice that had been infected with either S. aureus or P. aeruginosa.
Sign up for the Live Science daily newsletter now
Get the world’s most fascinating discoveries delivered straight to your inbox.
Given that the study was conducted in rodents in the lab, "moving on into a human system is, you know, that huge next step," said Thien-Fah Mah, a professor and director of the Microbiology Graduate Program at the University of Ottawa who was not involved in the research. And, as with any new drug-like molecules, more studies will be needed to pin down what dose and administration route would be the safest and most effective in people, Mah told Live Science.
But given that most bacterial species use this defense tactic, taking aim at hydrogen sulfide production could be a "true game changer" in the fight against antibiotic resistance, Mah wrote in a commentary, also published June 10 in the journal Science.
Long road to discovery
The road to the current study began years ago, when a 2007 report in the journal Cell introduced the idea that all bactericidal antibiotics might trigger cell death in the same way, Mah said. "At that point ... it kind of blew the lid off of what all of us were thinking," because each class of bactericidal antibiotics targets different parts of the bacterial cell, so it's counterintuitive to think they work the same way to ultimately kill microbes, she said.
For example, some bactericidal drugs target a cell's outer wall, while others disrupt its protein-building factory, the ribosome. But the 2007 paper suggested that, after hitting their primary targets, all these drugs trigger a common secondary effect: They push bacteria to produce "reactive oxygen species," also known as free radicals, highly reactive molecular wrecking balls that can seriously damage DNA and proteins if not promptly defused.
Following this work, Nudler and his colleagues discovered one of bacteria's natural defense mechanisms against reactive oxygen species: hydrogen sulfide. According to their report, published in 2011 in the journal Science, the team scoured the genomes of hundreds of bacteria and found that they shared common genes that code for hydrogen sulfide-producing enzymes, with S. aureus and P. aeruginosa primarily using CSE. They reported that hydrogen sulfide boosted production of antioxidant enzymes in the bacteria, which transform free radicals into non-toxic molecules, while also suppressing the production of reactive oxygen species.
They also found that deleting or disabling the enzymes in bacteria made them "highly sensitive" to a wide range of antibiotics. These sensitized bacteria died from oxidative stress caused by a buildup of reactive oxygen species. At that point, the team wanted to find "inhibitors" that could bind and disable bacterial enzymes in an infected person.
Related: 12 amazing images in medicine
"If we combined those inhibitors with antibiotics … we could make those antibiotics more potent," Nudler told Live Science. However, "it was very tricky to find those inhibitors targeting these enzymes that were specific to bacteria," he noted.
Mammalian cells also produce hydrogen sulfide, meaning human cells also rely on the compound; in humans, hydrogen sulfide acts as a signalling molecule and interacts with many tissues, from the brain to smooth muscle. Both human cells and bacterial cells use CSE to make hydrogen sulfide, but the human and bacterial CSE come in slightly different flavors. The team wanted to find molecules that would show a strong preference for the bacterial CSE, both to ensure that the chemicals would be potent against bacteria and to avoid any unintended side effects on mammalian cells.
To do so, they extensively studied the structure of human, bacterial and other versions of CSE to find an attractive target for their molecules to latch onto. Ultimately, they found a "nice pocket" on the bacterial CSE that a small molecule could slip into and change the activity of the enzyme, Nudler said.
"What they've done is, they actually identified something that's unique to the bacterial enzyme and is not present in the human enzyme … so this is specific for bacteria," Mah said. Having found a bull's-eye to aim at, the team set to work crafting their weapons. They ran a virtual screen of about 3.2 million commercially available small molecules to determine which would fit into their chosen pocket. Three stood out as promising choices and made it to the next round of experiments.
By tamping down hydrogen sulfide production, the inhibitors not only boosted the effects of antibiotics against the bugs but also suppressed a phenomenon known as "bacterial tolerance."
Unlike antibiotic resistance, in which bacteria evolve in ways that make them less susceptible to drugs, tolerance describes when bacteria turn down their metabolisms in the face of stress and enter a somewhat dormant state. In this state, the cells stop multiplying and reduce their energy use. Because many antibiotics work by causing bacteria to short-circuit while multiplying, tolerance keeps the bacteria alive until the antibiotics are gone. This means some bacteria cells can linger even after an infected person completes a full course of antibiotics, and if their immune system isn't equipped to deal with the leftovers, chronic infection can set in, Nudler said.
But in their experiments, the authors found that the inhibitors stopped many bacteria from switching into this protective state. "We demonstrate that hydrogen sulfide, clearly, makes a huge impact on tolerance," Nudler said. Currently, "there is no drug specifically targeting … this tolerance phenomenon," he added, suggesting this could be a new avenue for treatment.
That said, "from a mechanistic point of view, it is still not entirely clear how inhibition of hydrogen sulfide leads to the various effects observed," said Dr. Dao Nguyen, an associate professor in the department of microbiology and immunology at McGill University in Montreal, who was not involved in the study. Echoing the sentiment, Nudler noted that he and his colleagues plan to further investigate the role of hydrogen sulfide in tolerance.
The team also needs to determine whether they need to tweak the molecules to make them optimally effective for humans, not just mice, and to determine the best route of administration, Nguyen said. "If the inhibitors could be developed into safe and effective drugs, one could imagine that they would be used in combination with existing antibiotics to treat ... chronic infections where current antibiotics are not very effective," she said.
Originally published on Live Science.
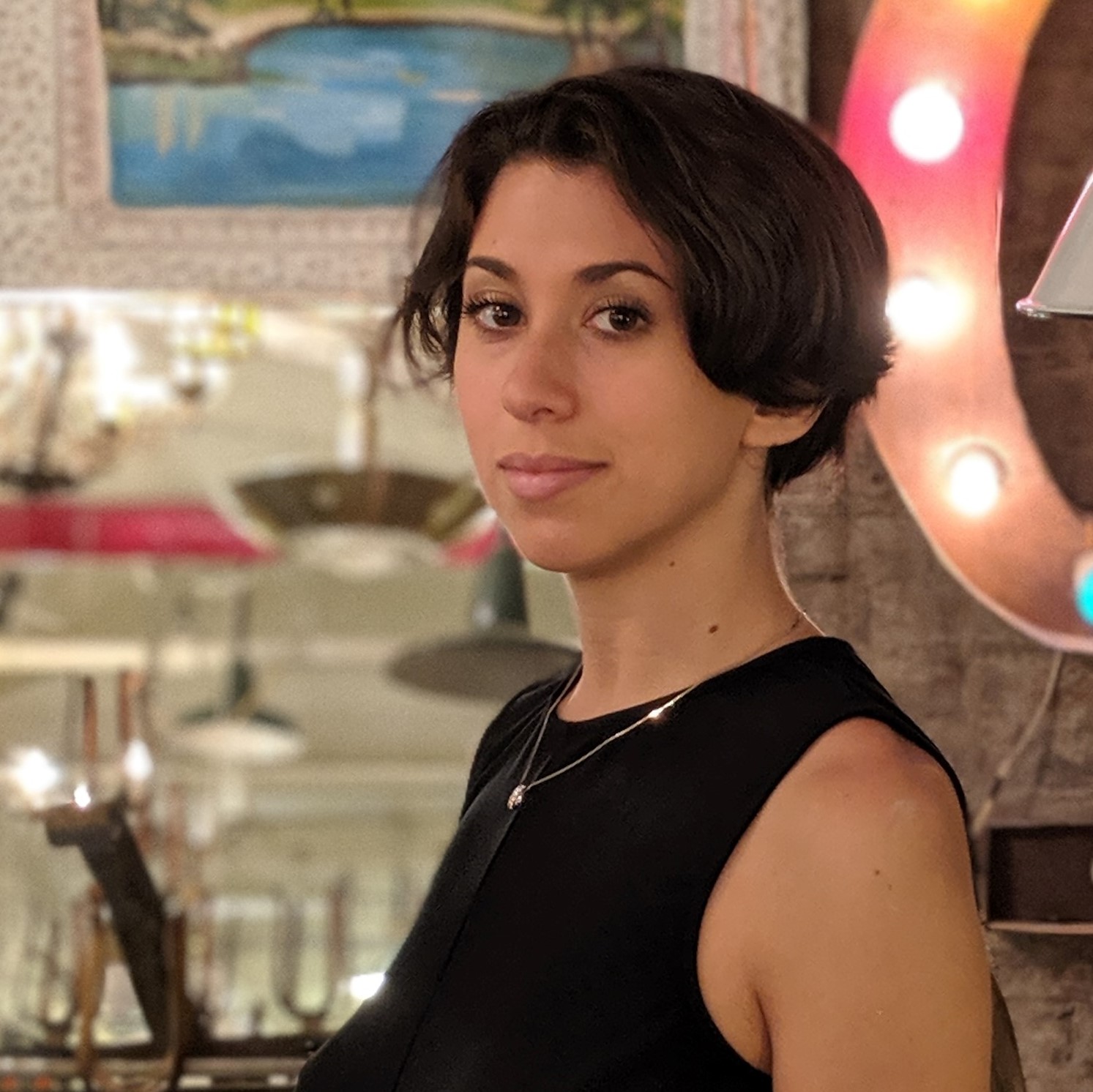
Nicoletta Lanese is the health channel editor at Live Science and was previously a news editor and staff writer at the site. She holds a graduate certificate in science communication from UC Santa Cruz and degrees in neuroscience and dance from the University of Florida. Her work has appeared in The Scientist, Science News, the Mercury News, Mongabay and Stanford Medicine Magazine, among other outlets. Based in NYC, she also remains heavily involved in dance and performs in local choreographers' work.