These 12 individuals have a rare genetic quirk that prevents 'self-eating' in cells
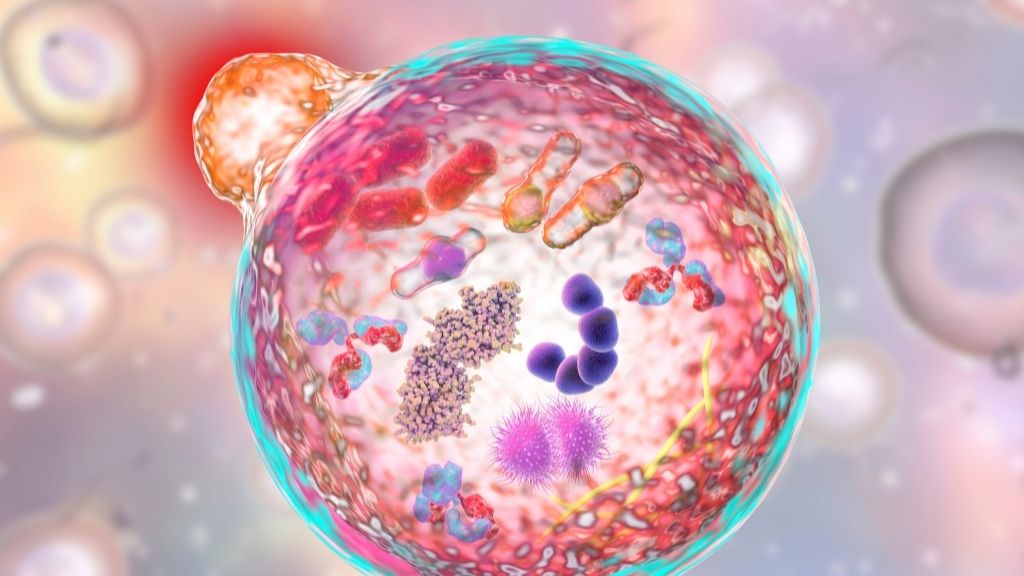
Scientists uncovered a rare genetic quirk in 12 people, from five different families, that leaves their cells unable to properly recycle their worn-out parts. Such mutations could be lethal, but these individuals have survived and instead live with neurodevelopmental conditions.
Normally, cells dispose of broken internal machinery, dysfunctional proteins, toxins and pathogens through a process called autophagy, which translates from Greek as "self-eating." In the process, cells package all their trash into special bags, called autophagosomes, which then fuse with the cell's garbage disposal, the lysosome. Lysosomes contain digestive enzymes that break down all the trash so that the component parts can be reused by the cell.
In humans, when autophagy goes awry, the subsequent buildup of cellular junk can contribute to various diseases, from neurodegenerative disorders to cancer, according to a 2020 report in the New England Journal of Medicine. This dysfunction can occur when mutations crop up in one of about 20 key genes involved in autophagy.
Related: 5 ways your cells deal with stress
And according to animal studies, if any of these 20 genes are severely impaired or completely disabled, it's usually impossible for the animal to survive. For instance, genetically modified mouse pups born without an essential autophagy gene called ATG7 die within 24 hours of birth, according to various reports. And deleting the same gene from adult mice causes them to die of infection or neurodegeneration within months, according to a 2014 report in the journal Cancer Discovery.
"The studies from mice suggest you can't live without them," meaning the 20 core genes, said senior author Robert Taylor, a professor of mitochondrial pathology at Newcastle University in England. "So, we thought that was the same in humans." But now, Taylor and his team have identified 12 people with defective ATG7 genes that leave them with little to none of the protein that the gene encodes, they reported June 23 in the New England Journal of Medicine (NEJM).
The ATG7 protein jumpstarts the process of building autophagosomes, the cell's special garbage bags, supposedly making it crucial to the entire autophagy process. The fact that the 12 identified individuals have survived, albeit with neurological disorders, "tells us something, that there is something that we don't know yet about autophagy biology that must be compensating for this process in humans," Taylor said.
Sign up for the Live Science daily newsletter now
Get the world’s most fascinating discoveries delivered straight to your inbox.
"An obvious question is what allows these patients to survive so long with greatly diminished autophagic capacity?" said Daniel Klionsky, a cell biologist and professor at the University of Michigan's Life Sciences Institute, who was not involved in the study. If other mechanisms do compensate for the lack of ATG7, the next step is to identify them and determine whether those mechanisms can be manipulated as a form of treatment for such genetic disorders, Klionsky told Live Science in an email.
Mysterious mutations
Since mutations in autophagy-related genes often have lethal consequences, "it is difficult to find an adequate number of patients to have meaningful results" when researching such genetic changes in humans, Klionsky noted. The fact that the team was able to find this number of people with ATG7 mutations "makes the findings more robust," he said.
The researchers found the first two study participants through a clinic that specializes in mitochondrial diseases, as some of their symptoms seemed consistent with mitochondrial conditions, Taylor said. The patients — two sisters whose respective ages were 28 and 18 — both showed mild-to-moderate learning difficulties, muscle weakness and a lack of coordination, known as ataxia, as well as hearing loss, eye abnormalities and facial dysmorphisms.
Brain scans taken of the elder sister revealed cerebellar hypoplasia, a condition where the cerebellum, located behind the brainstem, fails to develop properly. This region of the brain is critical for coordinating movement. The corpus callosum, a bundle of nerves that connects the two halves of the brain, also appeared unusually thin toward the back of the brain.
In seeing the shared symptoms between the sisters and striking brain scans from the eldest, "We realized that the best way to approach this was genetically, and we took it from there," Taylor said. The team found that both sisters carried recessive mutations in the ATG7 gene that greatly reduced or eliminated its ability to make ATG7 protein.
"And we thought, 'This can't be right,'" given the disastrous effects of ATG7 deficiencies seen in mice, Taylor said. "And yet we were able to show ... that actually, we can't detect ATG7 in the muscle [or] in the cells that we've grown from the first family." Hoping to better understand these counterintuitive results, the team went in search for more individuals with similar ATG7 mutations to the sisters.
Related: Genetics by the numbers: 10 tantalizing tales
"You can't make a compelling case with one family," whereas finding several families with the same combination of genetic mutations and clinical symptoms would strengthen their findings, Taylor said. "Then you start to kind of do the detective work that puts all this together and makes you think, 'We're onto something.'"
So the study's lead author Jack Collier, then a doctoral student in Taylor's lab, used an online tool called GeneMatcher to find the 10 other patients in the research’s cohort of 12. The tool, developed with support from the Baylor-Hopkins Center for Mendelian Genomics, is intended to connect patients, researchers and clinicians with an interest in the same genes.
Through GeneMatcher, the team identified four more families, located in France, Switzerland, Germany and Saudi Arabia. The family members who carried ATG7 mutations ranged from 6 weeks to 71 years in age and showed a similar suite of neurological symptoms, although the severity of the symptoms varied between individuals. In general, the patients showed neurodevelopmental deficits, facial dysmorphisms and ataxia. One or more patients from each family also underwent brain scans, and like the first patient, had underdeveloped cerebellums and thin corpus callosums.
In all but the first two patients, the team found some residual ATG7 protein in sampled muscle cells, as well as in fibroblasts — cells in connective tissue that secrete collagen — that the team grew from patient samples. And even in the first two sisters, some proteins involved in autophagy still cropped up in their cells, albeit in very low quantities. This hinted that the individuals' genetic mutations didn't completely suppress autophagy.
Looking closer at the mutations, the researchers found that each patient carried slightly different variations of the ATG7 gene, Taylor said. A mutation occurs when one DNA building block is swapped out for another, and the location of this swap along the DNA strand determines how the mutation will change the resulting protein. Using computer models, the team mapped out where all the patients' mutations appeared and found a general theme: The mutations cropped up in highly conserved portions of the DNA sequence, meaning they're usually the same across a wide range of organisms, from yeast to mice to humans.
In fact, the ATG7 gene is highly conserved in all eukaryotic cells — the complex cells that make up animals, plants, fungi and protists. Because of this, the team could test how mouse and yeast cells were affected by the mutations seen in the human patients. In lab dish studies, the mutations reduced or eliminated autophagy in both mouse and yeast cells, strengthening the case that the same was happening in the human patients' bodies.
"It is difficult to carry out experiments with humans," Klionsky said. "Certainly, the inclusion of data from mouse and yeast studies makes the results much stronger."
Related: How to speak genetics: A glossary
That said, many questions about these mysterious mutations remain unanswered. Namely, how do people survive when their cells can't "eat themselves" through the usual means?
The cells must be dealing with dysfunctional proteins and broken machinery to some degree, "because accumulations of cellular 'junk' was not observed," Ian Ganley, a principal investigator whose lab studies autophagy at the University of Dundee in Scotland, wrote in a commentary in NEJM. This indicates that some other mechanism fills in for the lack of ATG7-related autophagy, Ganley wrote.
Identifying such mechanisms will be key to developing treatments to syndromes where autophagy is impaired, whether due to a genetic quirk as described in the new study or in neurodegenerative diseases like Alzheimer's, he added. Such treatments could include drugs that boost the activity of these alternative mechanisms, helping cells to rid themselves of junk more efficiently, Taylor said. Another option could be gene therapy, where working copies of faulty autophagy genes are inserted into the genome to replace the mutant versions, Klionsky said.
For now, Taylor and his team plan to run experiments in cells to better understand how the mutations impact specific tissues, such as the brain and muscles, Taylor said. To this end, the team has already begun developing a line of induced pluripotent stem cells — those that can mature into any cell in the body — from patient samples. With those stem cells, the researchers can create fibroblasts and brain cells to see how the mutation impacts those cells.
"At the moment, we're still trying to understand some of the biology but want to do that in a relevant system," Taylor said. Only then can the team tackle the question of which potential treatments might be able to boost autophagy when it falters.
Originally published on Live Science.
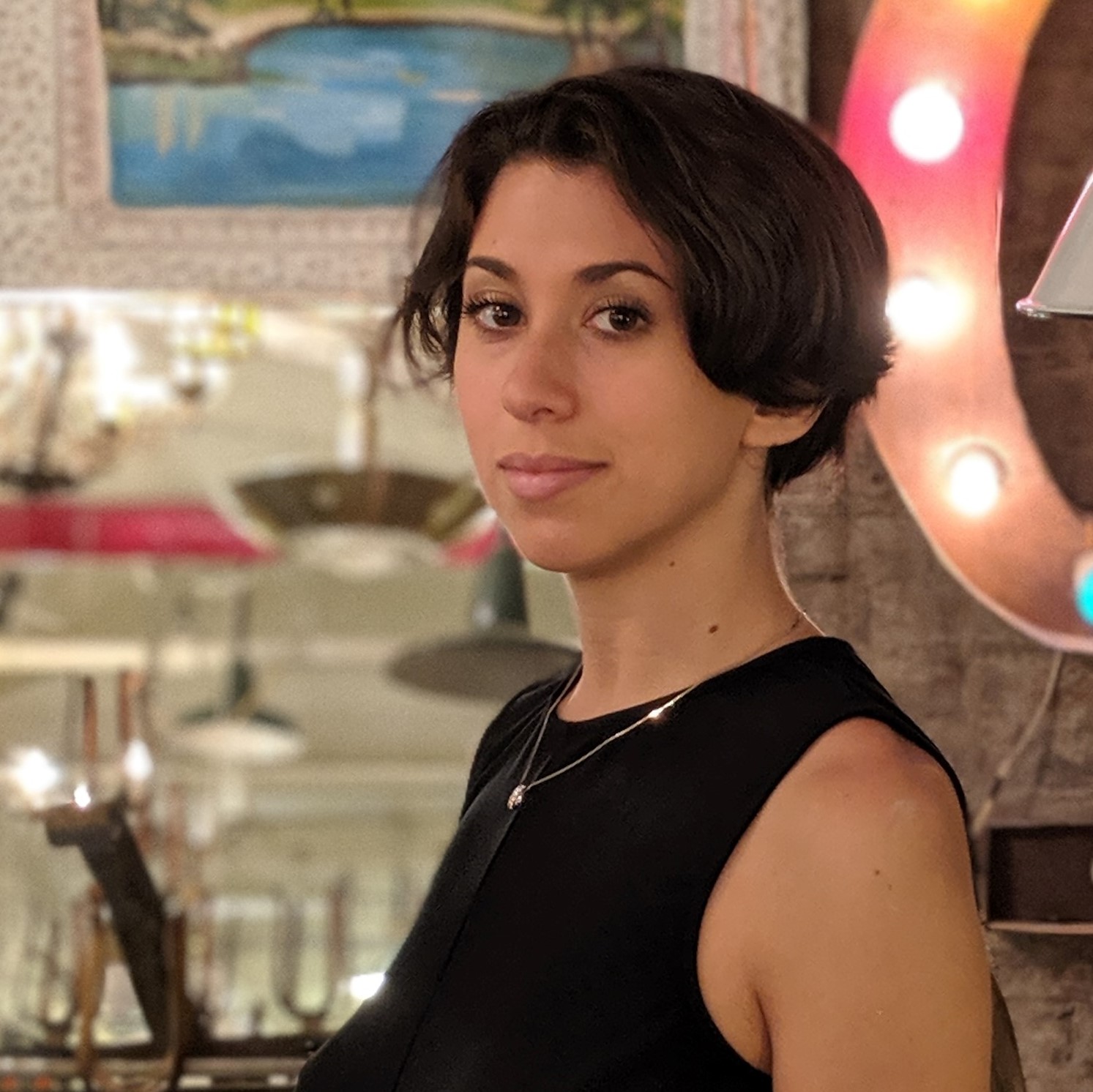
Nicoletta Lanese is the health channel editor at Live Science and was previously a news editor and staff writer at the site. She holds a graduate certificate in science communication from UC Santa Cruz and degrees in neuroscience and dance from the University of Florida. Her work has appeared in The Scientist, Science News, the Mercury News, Mongabay and Stanford Medicine Magazine, among other outlets. Based in NYC, she also remains heavily involved in dance and performs in local choreographers' work.