Time Crystals to Tetraquarks: Quantum Physics in 2017
Quantum Physics in 2017
The year 2017 was wild and unpredictable. And what science is better-suited for a weird year than quantum physics?
This year ushered in astonishing quantum discoveries from all corners — deep-buried neutrino labs in Antarctica, quantum-computing labs at major universities and even thunderstorms rumbling over Japan. From time crystals to the elusive tetraquark, here are 15 of the most amazing quantum discoveries of the past 12 months.
A simple quantum computer
Quantum computers are coming, and they're bringing some capabilities that will outstrip those of modern computers. But actually building them remains a complicated proposition — past efforts relied on laboratory lasers or other rarified equipment. Now, researchers at the University of Sussex have produced a . In theory, the quantum computer could operate in far less extreme conditions and at a far larger scale than current models.
A time crystal
Time crystals sound like talismans from a fantasy novel, but they're actually real quantum objects, generated for the first time in 2017. A time crystal is a unique state of matter, a group of ions lashed together with quantum links such that they shake, or oscillate, together at a repeating period. As long as the conditions they need to exist remain, time crystals become locked into a single moment in time; no matter how much energy gets pumped into them, they don't heat up or increase in entropy — effectively suspending the laws of thermodynamics as they're typically understood. [The 18 Biggest Unsolved Mysteries in Physics.]
Ultracold particles
Quantum physics is (more or less) the science of what happens when very small things interact without the interference of larger, outside forces and energies. So in order to make many quantum-mechanical experiments work, researchers need to cool small groups of particles way, way down — to the point where heat doesn't let atoms jiggle. Researchers have long used lasers to slow particles down, sapping their energy. But a new technique, using magnetic fields to trap molecules in place before laser-slowing them, takes that method a step further and works for many more kinds of molecule. In an early experiment with this technique, researchers at Imperial College London cooled a molecule of calcium monofluoride to 50 microkelvin — 50 millionths of a degree above absolute zero.
Neutrino symmetries
New results from IceCube Neutrino Observatory — demonstrations in a laboratory buried 0.9 miles (1.45 kilometers) under the ice surface in Antarctica, which studies difficult-to-detect particles — suggest that there is a strange symmetry in the masses of neutrinos. Neutrinos divide into three flavors: muon, tau and electron. And their masses divide into three "eigenstates," or, informally, species. One species appears to be made up of mostly electron neutrinos and a second species is made up of an almost equal mix of all three flavors. But the new evidence suggests that the third species is made up of an equal split of muon and tau neutrinos — and that's a symmetry scientists still can't explain, suggesting there might still be hidden rules governing neutrinos that physicists have yet to uncover.
Doubly charmed particle
An experiment at the atom-smashing Large Hadron Collider (LHC), a 17-mile-long (27 km) underground ring near Geneva, revealed a baryon — or three-quark particle — made up of two heavy "charm" quarks and one lighter "up" quark. When the elusive, so-called Xi-cc-plus-plus particle, a distant cousin of more common baryons like neutrons and protons, appeared, it validated the predictions of the Standard Model, the reigning theory that explains subatomic particles. The theoretical physicists Jonathan Rosner and Marek Karliner had developed a method for estimating the mass of as-yet-undiscovered baryons, and this experiment came very close to their prediction, demonstrating that it worked.
Explosive quantum secret
When Rosner and Karliner's method turned out to work, they were able to apply it in other contexts. One notable example: they CKCK discovered it was potentially possible to fuse two extra-heavy, "bottom" quarks and form a nucleon. That mindboggling "quarksplosion" would release an enormous amount of energy — 7.6 times the energy of the individual hydrogen fusion reactions that take place inside atom bombs. The discovery scared Karliner enough, he told Live Science, that he considered keeping it a secret, until he made sure that there was no way to assemble enough of the material involved to build a weapon.
Elusive tetraquark
Rosner and Karliner's technique for determining the mass of theoretical particles also allowed them to predict that the "holy grail" hadron, a tetraquark — a particle made up of four quarks, not the three found in baryons — could really exist in its purest form. They even predicted its mass. Their research points the way toward future experiments at the LHC, which should sooner or later reveal a real-life tetraquark on Earth. [7 Strange Facts About Quarks]
Lightning-bolt particle accelerators
Since 1925, researchers have suspected that the electron cascades of lightning bolts might trigger atom-splitting processes. But for the first time in 2017, researchers proved that lightning is a giant antimatter factory. The flashes of lightning inside thunderstorms, they showed, unleash a chain of events that cause neutrons to split away from ambient nitrogen and oxygen atoms. This process leaves behind unstable, radioactive isotopes — and showers of neutrinos and antimatter, which are detectable from the ground, due to telltale gamma-ray flashes as the antimatter encounters matter and annihilates. [Infographic: How Lightning Works]
Long-distance quantum entanglement
The Chinese Micius satellite began sending and receiving interesting quantum particles to and from Earth this summer. The first trick? Shattering the record for separating entangled particles. Micius sent a pair of entangled — or quantum-linked — protons to base stations on Earth separated from one another by a distance of 747.5 miles (1,203 km), the first step toward building secure encryption for a quantum internet.
Quantum teleportation
Within a month of the Micius entanglement announcement, the Micius team announced another achievement: quantum teleporting a packet of information from a proton on an Earth base station to a proton up on the satellite in orbit. Again, this is the kind of long-distance information transfer that future quantum networks will have to reliably achieve.
Sign up for the Live Science daily newsletter now
Get the world’s most fascinating discoveries delivered straight to your inbox.
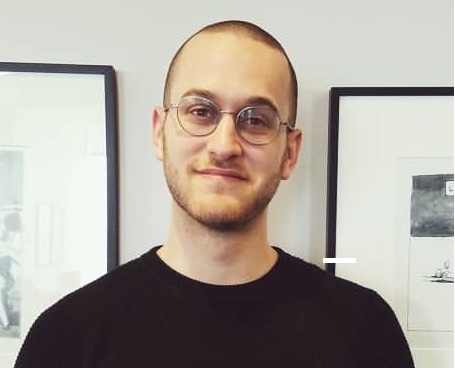