7 Strange Facts About Quarks
Teensy Particles
Quarks are particles that are not only hard to see, but pretty much impossible to measure. These teensy-tiny particles are the basis of subatomic particles called hadrons. With every discovery in this field of particle physics in the past 50 years, however, more questions arise about how quarks influence the universe's growth and ultimate fate. Here are seven strange facts about quarks.
Emerged just after Big Bang
The first quarks appeared about 10^minus 12 seconds after the universe was formed, in the same era where the weak force (which today is the basis for some radioactivity) separated from the electromagnetic force. The antiparticles of quarks appeared around the same time.
Discovered in an atom smasher
A mystery arose in the 1960s when researchers using the Stanford Linear Accelerator Center found that the electrons were scattering from each other more widely than calculations suggested. More research found that there were at least three locations where electrons scattered more than expected within the nucleon or heart of these atoms, meaning something was causing that scattering. That was the basis for our understanding of quarks today.
Mentioned by James Joyce
Murray Gell-Mann, the co-proposer for the quark model in the 1960s, drew inspiration for the spelling from the 1939 James Joyce book "Finnegan's Wake," which read: "Three quarks for Muster Mark! / Sure he has not got much of a bark / And sure any he has it's all beside the mark." (The book came out well before quarks were discovered and so their name has always been spelled in this way.)
Come in flavors
Physicists refer to the different types of quark as flavors: up, down, strange, charm, bottom, and top. The biggest differentiation between the flavors is their mass, but some also differ by charge and by spin. For instance, while all quarks have the same spin of 1/2, three of them (up, charm and top) have charge 2/3, and the other three (down, strange and bottom) have charge minus 1/3. And just because a quark starts out as a flavor doesn't mean it will stay that way; down quarks can easily transform into up quarks, and charm quarks can change into strange quarks. [Read more about quark flavors]
Tricky to measure
Quarks can't be measured, because the energy required produces an antimatter equivalent (called an antiquark) before they can be observed separately, among other reasons, according to a primer from Georgia State University. The mass of quarks is best determined by techniques such as using a supercomputer to simulate the interactions between quarks and gluons, with gluons being the particles that glue quarks together.
Teach us about matter
In 2014, researchers published the first observation of a charm quark decaying into its antiparticle, providing more information about how matter behaves. Because particles and antiparticles should destroy each other, one would think the universe should just have photons and other elementary particles. Yet antiphotons and antiparticles still exist, leading to the mystery of why the universe is made mostly of matter and not antimatter.
May set the universe's fate
Nailing down the mass of the top quark could reveal to researchers one of two ghastly scenarios: that the universe could end in 10 billion years, or that people could materialize out of nowhere. If the top quark is heavier than expected, energy carried through the vacuum of space could collapse. If it's lower than expected, an unlikely scenario called "Boltzmann brain" could see self-aware entities come out of random collections of atoms. (While this isn't a part of the Standard Model, the theory – framed as a paradox – goes that it would be more likely to see organized groups of atoms as the random ones observed in the universe.)
Sign up for the Live Science daily newsletter now
Get the world’s most fascinating discoveries delivered straight to your inbox.
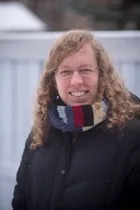
Elizabeth Howell was staff reporter at Space.com between 2022 and 2024 and a regular contributor to Live Science and Space.com between 2012 and 2022. Elizabeth's reporting includes multiple exclusives with the White House, speaking several times with the International Space Station, witnessing five human spaceflight launches on two continents, flying parabolic, working inside a spacesuit, and participating in a simulated Mars mission. Her latest book, "Why Am I Taller?" (ECW Press, 2022) is co-written with astronaut Dave Williams.