
Wacky Physics: Why Do Particles Have Flavors?
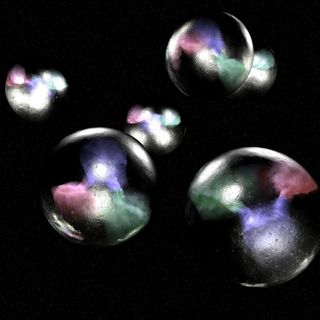
In this regular series, LiveScience explores some of the wildest, weirdest parts of our universe, from quantum oddities to hidden dimensions.
The building blocks of matter — fundamental particles — come in many more flavors than the basic few that make up the atoms we're familiar with.
Flavor is the name scientists give to different versions of the same type of particle. For instance, quarks (which make up the protons and neutrons inside atoms) come in six flavors: up, down, top, bottom, strange and charm. Particles called leptons, a category that includes electrons, also come in six flavors, each with a different mass.
But physicists are baffled as to why flavors exist at all, and why each flavor has different characteristics.
"This is known as the flavor problem," said JoAnne Hewett, a theoretical physicist at the SLAC National Accelerator Laboratory in Menlo Park, Calif. "Why are there so many flavors? Why do we have six types of quarks and six types of leptons, and why do they have the different masses that they do? We don't have a clue." [Graphic: Nature's Tiniest Particles Explained]
Changing flavor
In the strange world of particle physics, the various flavors of quarks and leptons are differentiated by their individual properties, including mass, charge and spin.
Sign up for the Live Science daily newsletter now
Get the world’s most fascinating discoveries delivered straight to your inbox.
For example, all quarks have the same spin (1/2), and three of them (up, charm and top) have charge 2/3, while the other three (down, strange and bottom) have charge minus 1/3. Each one has a unique mass.
What's even stranger is that particles are able to switch from one flavor to another. For example, down quarks can easily turn into up quarks, and charm quarks can turn into strange quarks, and so on. While some transitions are more common than others, in theory, most flavors of quark can transition into most other flavors.
"We don't know what's inside a quark," said Michael Peskin, another particle physicist at SLAC. "We think it's the similarity or dissimilarity of internal structure that makes it hard or easy to make these transitions."
And while particles do come in many flavors, our universe is preferentially made up of just a few.
The elements in the periodic table, such as carbon, oxygen and hydrogen, are composed of protons, neutrons and electrons. Protons and neutrons, in turn, contain just up and down quarks; top and bottom, charm and strange quarks are nary to be found.
Same goes for leptons: While electrons abound, some of the other flavors, such as muons and taus, are rarely found in nature.
"They existed in the very early fractions of a second of the universe and then they decayed away," Hewett told LiveScience, referring to the rare particle flavors. "They don’t exist really in everyday life."
Other mysteries
Besides searching for the origin of flavor, physicists studying these topics also hope to learn about related mysteries, such as matter's weird twin, antimatter. Every particle is thought to have an antimatter partner, with the same mass, but the opposite charge.
Yet physicists think there should be a lot more antimatter in the universe than there is, and flavor physics may help to explain this "loss" of antimatter.
"There's a matter-antimatter asymmetry in the universe, in the sense that the universe is made out of matter and there's no antimatter observed today, but in the Big Bang, matter and antimatter were created in equal amounts," Hewett said. "So what happened to all the antimatter? We think this is related to flavor physics."
When a particle and its antimatter partner meet, they annihilate each other to become pure energy. Most of the matter and antimatter particles created at the beginning of the universe are thought to have destroyed each other, leaving a small amount of matter left over that became the stars and galaxies we see today.
Physicists think that differences in the way matter decays compared with antimatter may explain why matter took longer to decay, and therefore survived. Researchers have observed some asymmetries in the decay rates of matter and antimatter, but these alone are not sufficient to explain the universe as we see it.
"You get a difference with these asymmetries, but it's about a billion times smaller than you need," Peskin said. "There have got to be some other new equations that we haven't seen the evidence for yet that also predict different kinds of matter-antimatter asymmetries."
Scientists are hoping that by studying the weird flavor behavior of particles, they might go further toward explaining matter's persistence after the Big Bang.
The intensity frontier
Researchers' best hope of getting to the bottom of particle flavor may lie in a slew of new experiments being proposed to tackle what's called the "intensity frontier."
In these experiments, researchers want to observe particles' transition from one flavor to another, and not just the common transitions, such as a down quark into an up quark, but more exotic switcheroos, such as the change of a bottom quark into a charm quark.
But to do this, scientists must increase the intensity, or number of particles produced, in their particle accelerators.
"We're looking for rare phenomena, so the way to observe that is to make many, many instances of it," said particle physicist Robert Tschirhart of the Fermi National Accelerator Laboratory in Batavia, Ill. "If you want to win the lottery, you've got to buy a lot of lottery tickets."
Tschirhart is the lead scientist for Project X, a Fermilab plan to build an extremely high-intensity particle accelerator that would look for rare flavor transitions.
"We would produce a very high flux of neutrinos and a very high flux of K mesons, which are unstable particles that have strange quarks inthem, and a very high flux of muons, which are unstable particles, heavier cousins of normal electrons," Tschirhart said. "It would be the biggest accelerator project in the U.S., it would be the highest intensity particle accelerator for particle physics."
Other ventures to build new high facilities for flavor physics are under way in Italy and Japan.
Furthermore, the world's largest particle accelerator, the Large Hadron Collider in Switzerland, has an experiment called LHCb devoted to searching for certain rare decays of particles called b mesons, which contain different flavored quarks.
Extra dimensions
Though scientists are generally stumped on where particles get their flavor, one theory does propose a fascinating, and bizarre, solution.
Particle flavors may be a symptom of an extra, hidden dimension of the universe beyond the three dimensions of space and one of time that we are used to. This concept, called warped dimensions, was pioneered by physicists Lisa Randall and Raman Sundrum.
"Maybe those different flavors are actually, in a funny way, different dimensions of space and time," Tschirhart said. "Maybe there's just one kind of flavor of quark and these different flavors we see — strange, charm, top, bottom — those are different geometrical features of space and time."
For those of us scratching our heads at the connection, Tschirhart reminds us that Einstein himself showed that mass is intimately connected with space and time when he drafted his general theory of relativity. According to the theory, gravity, which is the attractive force of mass, is really a curvature of space-time.
We see the six different quarks as having six different masses, but perhaps they actually have the same mass, but are located at different spots in the extra dimension, causing them to appear differently.
Though the theory may sound fantastical, it makes some concrete predictions about how certain particles decay and transition between flavors. Researchers hope that new experiments that push the intensity frontier may be able to measure some of these decays and possibly validate or invalidate this and other theories.
"I feel like the experiments we have in front of us right now have the best chance of making the biggest steps in terms of answering these questions," Hewett said. "Intensity frontier experiments are just really now getting to the levels of intensity where they have a chance of answering them."
You can follow LiveScience senior writer Clara Moskowitz on Twitter @ClaraMoskowitz. For more science news, follow LiveScience on twitter @livescience.