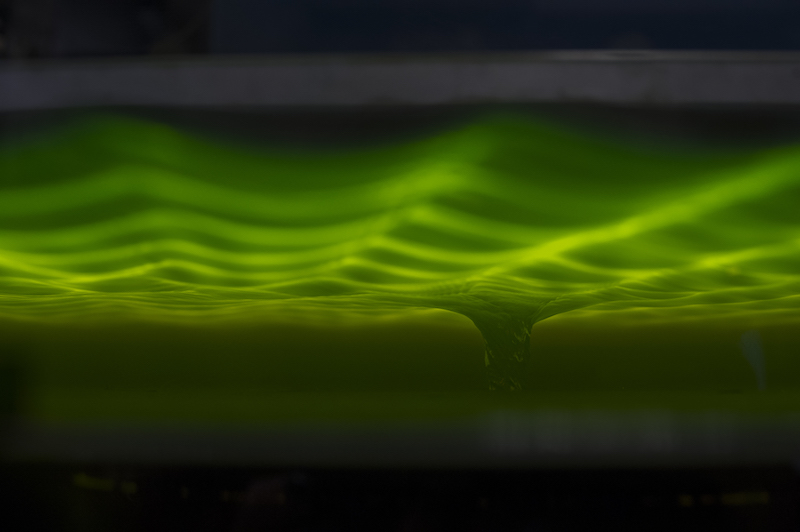
Mathematicians and scientists have simulated the region around a black hole using waves as they circled a drain in a simple tub of water.
The new simulation has, for the first time, confirmed a long-proposed theory, called superradiance, about how black holes wind down, said study co-author Silke Weinfurtner, a mathematician at the University of Nottingham in England.
"It turns out, waves in our setup follow the same mathematics, if you will, as small fluctuations around black holes," Weinfurtner told Live Science. "For little ripples in the system, this looks like an analogue of a rotating black hole." [Science Fact or Fiction? The Plausibility of 10 Sci-Fi Concepts]
Black-hole spin
Black holes, or incredibly massive and dense celestial objects from which not even light can escape, can be completely described by three characteristics: their mass, their charge and their spin or angular momentum. Black holes gobble up any objects that pass beyond their event horizon; not even gravity can escape their clutches. But what happens on the outskirts of a black hole's event horizon has been a little less clear.
Black holes can also die. One way these ultramassive objects dissolve is through a phenomenon known as Hawking radiation. This concept, first proposed by physicist Stephen Hawking, states that quantum radiation can leak from the edges of a black hole through a process known as quantum tunneling.
But in the past century, many physicists, including renowned mathematical physicist Roger Penrose, have proposed another way in which black holes could essentially wind down: Waves dragged around the periphery of the black hole without crossing its event horizon might gain angular momentum as the black hole itself starts spinning more slowly.
"Usually, when you send a wave toward an object, it will lose part of its energy — its energy will be absorbed by the object or scattered," said study co-author Mauricio Richartz, a physicist at the Federal University of ABC in Brazil. "With superradiance, it's the other way around: The wave approaches the object, and instead, it gains energy."
Sign up for the Live Science daily newsletter now
Get the world’s most fascinating discoveries delivered straight to your inbox.
One scientist even proposed that a similar mechanism might apply to electromagnetic waves as they approach a spinning cylinder. But there was no way to test it because the cylinder would have to rotate at nearly the speed of light to produce detectable levels of superradiance, Richartz said.
Big tub of water
But Weinfurtner and her colleagues thought there might be a simpler way to test the idea — by using water and waves. Weinfurtner first tried simple simulations using a bucket with a hole in the bottom, but that just led to flooding.
For the new study, which was published June 14 in the journal Nature Physics, the group used a more sophisticated version of a bathtub. The team used a massive, 9.8-foot-long (3 meters) tub with a drain in the center. They put fluorescent green dye in the water. Above the tub they placed light-detecting sensors that detect waves on the boundary between the water and the air, which, in turn, measures changes in wave speed in the water. A special piece of paper with small holes punched into it was also used to track fluid flow in the area around the vortex. As waves approached the circling "black hole" of the drain, they were deflected with about 14 percent more amplitude, or height to the waves, meaning they had actually gained energy.
The new findings show that the phenomenon of superradiance is surprisingly robust, even when conditions are not ideal, Weinfurtner said.
"What if you don't have a strict horizon? What if something can escape again?" Weinfurtner said.
(Obviously, lots of things can escape from a draining tub of water, unlike from a true black hole.)
It turned out that even these imperfect systems demonstrated the phenomenon.
"By extending an old water-wave analogue experiment in a technically challenging and imaginative way, Silke Weinfurtner and her colleagues have created what seems to be the first laboratory demonstration of the fundamental phenomenon of superradiance," Michael Berry, a physicist at the University of Bristol who was not involved in the work, wrote in an email to Live Science. [The Strangest Black Holes in the Universe]
Dark matter and astrophysical questions
The new method could also be a great testing environment for learning more about the mysterious celestial objects, said Luis Lehner, a physicist at the Perimeter Institute in Canada who was not involved in the study.
"Black holes are still very enigmatic objects," so being able to study some of their behavior in a controlled lab setting will help provide further intuition about them, Lehner added.
The findings could also help constrain some models of dark matter, the mysterious material that exerts a gravitational pull and makes up most of the universe's mass, yet doesn't interact with light, Lehner said.
In one type of model, for instance, dark matter is made up of a massive field. For some parameters, the interaction of this dark-matter field would significantly slow the angular rotation of the black hole via superradiance.
"Thus, measurements of black-hole spins can be used to constrain these models of dark matter," Lehner said.
Originally published on Live Science.
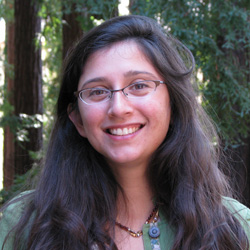
Tia is the managing editor and was previously a senior writer for Live Science. Her work has appeared in Scientific American, Wired.com and other outlets. She holds a master's degree in bioengineering from the University of Washington, a graduate certificate in science writing from UC Santa Cruz and a bachelor's degree in mechanical engineering from the University of Texas at Austin. Tia was part of a team at the Milwaukee Journal Sentinel that published the Empty Cradles series on preterm births, which won multiple awards, including the 2012 Casey Medal for Meritorious Journalism.