Photoelectric Effect: Explanation & Applications
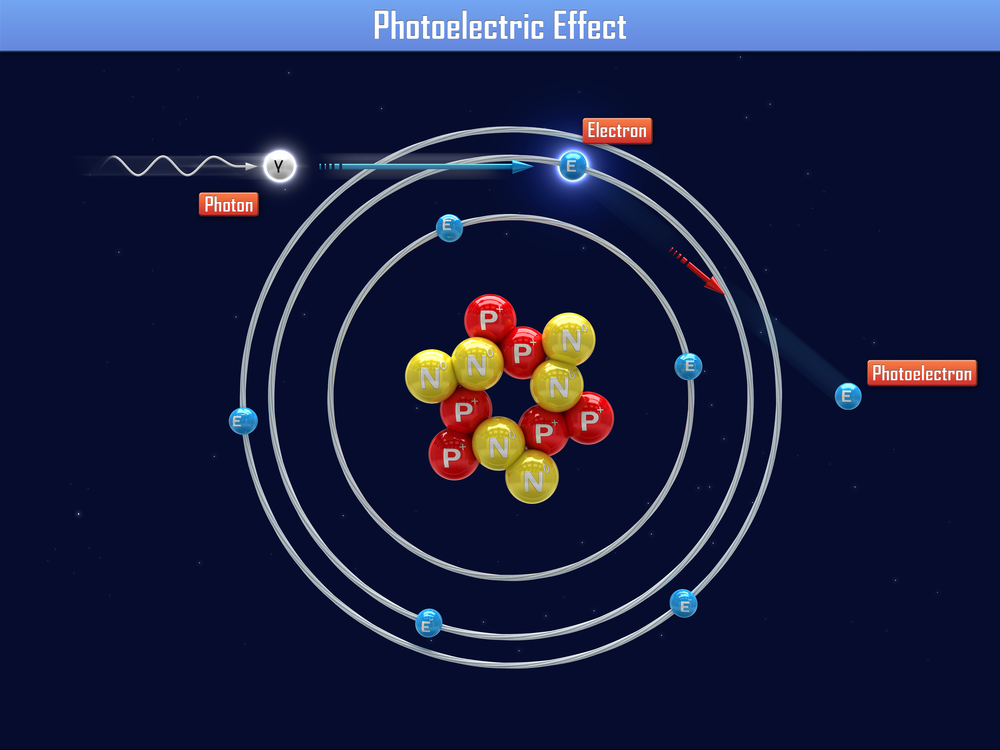
The photoelectric effect refers to what happens when electrons are emitted from a material that has absorbed electromagnetic radiation. Physicist Albert Einstein was the first to describe the effect fully, and received a Nobel Prize for his work.
What is the photoelectric effect?
Light with energy above a certain point can be used to knock electrons loose, freeing them from a solid metal surface, according to Scientific American. Each particle of light, called a photon, collides with an electron and uses some of its energy to dislodge the electron. The rest of the photon's energy transfers to the free negative charge, called a photoelectron.
Understanding how this works revolutionized modern physics. Applications of the photoelectric effect brought us "electric eye" door openers, light meters used in photography, solar panels and photostatic copying.
Discovery
Before Einstein, the effect had been observed by scientists, but they were confused by the behavior because they didn't fully understand the nature of light. In the late 1800s, physicists James Clerk Maxwell in Scotland and Hendrik Lorentz in the Netherlands determined that light appears to behave as a wave. This was proven by seeing how light waves demonstrate interference, diffraction and scattering, which are common to all sorts of waves (including waves in water.)
So Einstein's argument in 1905 that light can also behave as sets of particles was revolutionary because it did not fit with the classical theory of electromagnetic radiation. Other scientists had postulated the theory before him, but Einstein was the first to fully elaborate on why the phenomenon occurred – and the implications.
For example, Heinrich Hertz of Germany was the first person to see the photoelectric effect, in 1887. He discovered that if he shone ultraviolet light onto metal electrodes, he lowered the voltage needed to make a spark move behind the electrodes, according to English astronomer David Darling.
Then in 1899, in England, J.J. Thompson demonstrated that ultraviolet light hitting a metal surface caused the ejection of electrons. A quantitative measure of the photoelectric effect came in 1902, with work by Philipp Lenard (a former assistant to Hertz.) It was clear that light had electrical properties, but what was going on was unclear.
According to Einstein, light is made up of little packets, at first called quanta and later photons. How quanta behave under the photoelectric effect can be understood through a thought experiment. Imagine a marble circling in a well, which would be like a bound electron to an atom. When a photon comes in, it hits the marble (or electron), giving it enough energy to escape from the well. This explains the behavior of light striking metal surfaces.
While Einstein, then a young patent clerk in Switzerland, explained the phenomenon in 1905, it took 16 more years for the Nobel Prize to be awarded for his work. This came after American physicist Robert Millikan not only verified the work, but also found a relation between one of Einstein's constants and Planck's constant. The latter constant describes how particles and waves behave in the atomic world.
Further early theoretical studies on the photoelectric effect were performed by Arthur Compton in 1922 (who showed that X-rays also could be treated as photons and earned the Nobel Prize in 1927), as well as Ralph Howard Fowler in 1931 (who looked at the relationship between metal temperatures and photoelectric currents.)
Applications
While the description of the photoelectric effect sounds highly theoretical, there are many practical applications of its work. Britannica describes a few:
Photoelectric cells were originally used to detect light, using a vacuum tube containing a cathode, to emit electrons, and an anode, to gather the resulting current. Today, these "phototubes" have advanced to semiconductor-based photodiodes that are used in applications such as solar cells and fiber optics telecommunications.
Photomultiplier tubes are a variation of the phototube, but they have several metal plates called dynodes. Electrons are released after light strikes the cathodes. The electrons then fall onto the first dynode, which releases more electrons that fall on the second dynode, then on to the third, fourth, and so forth. Each dynode amplifies the current; after about 10 dynodes, the current is strong enough for the photomultipliers to detect even single photons. Examples of this are used in spectroscopy (which breaks apart light into different wavelengths to learn more about the chemical compositions of star, for example), and computerized axial tomography (CAT) scans that examine the body.
Other applications of photodiodes and photomultipliers include:
- imaging technology, including (older) television camera tubes or image intensifiers;
- studying nuclear processes;
- chemically analyzing materials based on their emitted electrons;
- giving theoretical information about how electrons in atoms transition between different energy states.
But perhaps the most important application of the photoelectric effect was setting off the quantum revolution, according to
Scientific American. It led physicists to think about the nature of light and the structure of atoms in an entirely new way.
Additional resources
Get the world’s most fascinating discoveries delivered straight to your inbox.
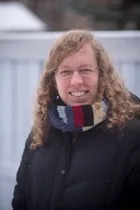
Elizabeth Howell was staff reporter at Space.com between 2022 and 2024 and a regular contributor to Live Science and Space.com between 2012 and 2022. Elizabeth's reporting includes multiple exclusives with the White House, speaking several times with the International Space Station, witnessing five human spaceflight launches on two continents, flying parabolic, working inside a spacesuit, and participating in a simulated Mars mission. Her latest book, "Why Am I Taller?" (ECW Press, 2022) is co-written with astronaut Dave Williams.