Twisters Pop Up in Weird 'Big Bang' Soup
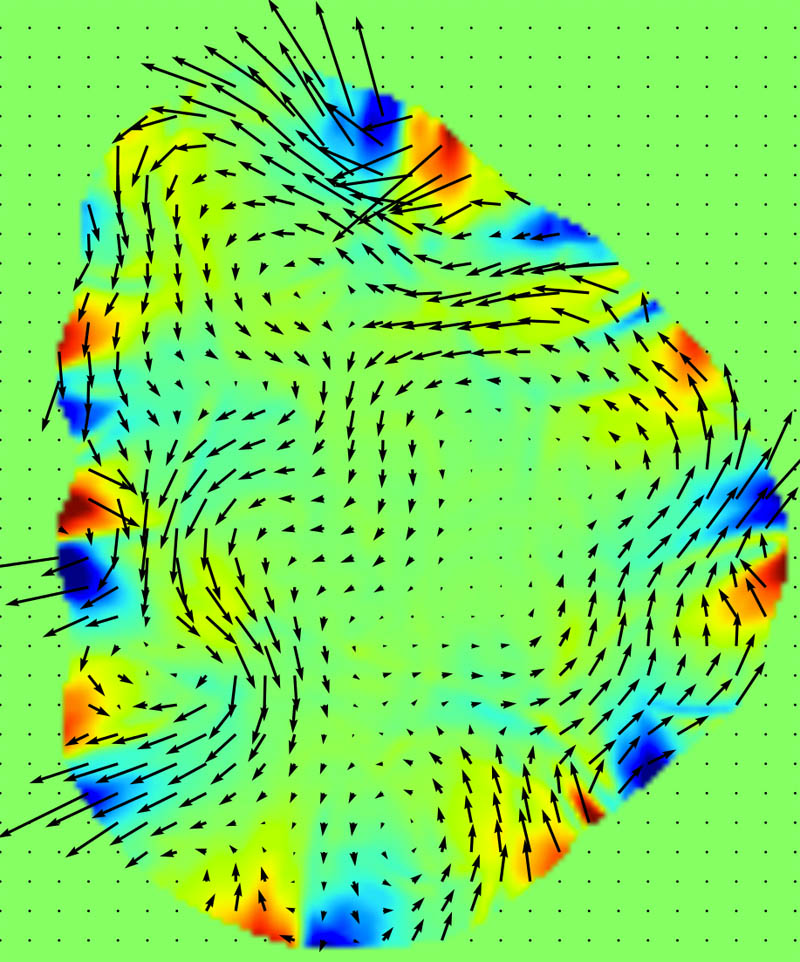
Smashing atoms together could produce a weird kind of fluid that makes whirlpools and rings, revealing secrets of some of the least-understood forces of nature that hold matter together, according to new research.
For years, physicists have been studying a substance called a quark-gluon plasma, a nearly frictionless fluid that permeated the universe at its inception, just after the Big Bang. The weird substance is a mix of the subatomic particles called quarks, which make up protons and neutrons, and gluons, which transmit the strong nuclear force that holds quarks together. [The 9 Biggest Unsolved Mysteries in Physics]
How this plasma behaves has been the subject of much interest because it can reveal the behavior of the strong nuclear force. "It's the least understood of the four fundamental forces," Mike Lisa, a professor of physics at The Ohio State University, told Live Science. (The other three forces are gravity, the weak nuclear force and electromagnetism.)
Melting matter
Scientists typically study this "soup" by actually creating it inside particle accelerators, where atomic nuclei traveling at near light speed collide into one another, sending temperatures skyrocketing to trillions of degrees Fahrenheit or hundreds to thousands of times hotter than the sun's core, the researchers said. Under those conditions, the protons and neutrons inside atomic nuclei melt, releasing quarks and gluons. The result is this quark-gluon plasma.
Recently, when simulating the quark-gluon plasma using a supercomputer, researchers found that the "soup" produced structures shaped like rings and vortices. Moreover, the viscosityof the fluid — its resistance to deforming — in the simulations was nearly as low as it could be and still followed the laws of quantum mechanics, the branch of physics that deals with extremely small particles. The simulated plasma was a superfluid, or a fluid with nearly zero friction, the researchers said.
Physicists had modeled the quark-gluon plasma as a fluid since 2005, but the latest computer simulations show the odd structures — such as vortices, rings and spokes — that pop up in such a fluid, said lead researcher Xin-Nian Wang, of Lawrence Berkeley National Laboratory in California. [The Mysterious Physics of 7 Everyday Things]
"In the beginning, people had simple models, a fireball-like plasma in high-energy interactions," Wang told Live Science. Basically, they assumed that the plasma would expand roughly evenly in a sphere.
Get the world’s most fascinating discoveries delivered straight to your inbox.
But the new work has found that the plasma will form whirlpools aligned with the beam of particles, as well as spokes. "There's more of a fiber-like geometry, fluctuating with a lot of lumps and valleys," Wang said.
One reason for the whirlpool structures is the transfer of momentum from the collisions, rather like cars that spin out after a head-on crash. The exact origins of the structures are still somewhat mysterious because the behavior was unexpected, according to Wang.
Vorticity measured
The researchers also found that the quark-gluon soup has a high "vorticity," meaning it is spinning really, really fast. Vorticity is a measure of how fast a section of a vortex spins relative to the whole. A tornado has a vorticity of about 0.001 (measured in inverse seconds, or occurences per second) — so a small region of a tornado takes about 17 minutes to complete a rotation, Lisa noted. The quark-gluon plasma has a vorticity of 10^21, so it makes one revolution in a tiny fraction of a second.
Wang's calculations show that the fluidic plasma in the vortices should generate lots of short-lived particles, called lambda baryons, with spins in the same "direction" (either "up" or "down"). This is because given plasma shapes produce certain kinds of particles.
If that doesn't happen, it means the calculations are off and the simulation needs work. "We're pretty confident about this model," he said.
However, experiments with tools such as the Relativistic Heavy Ion Collider (RHIC) will confirm if Wang and his team's simulations are correct, said Lisa, who has worked on both Brookhaven National Laboratory's RHIC in New York and the Large Hadron Collider at CERN (the European Organization for Nuclear Research).
"It's a whole new area," Lisa said. "The study of vorticity in these collisions is brand-new."
The study appears in the Nov. 1 issue of the journal Physical Review Letters.
Original article on Live Science.
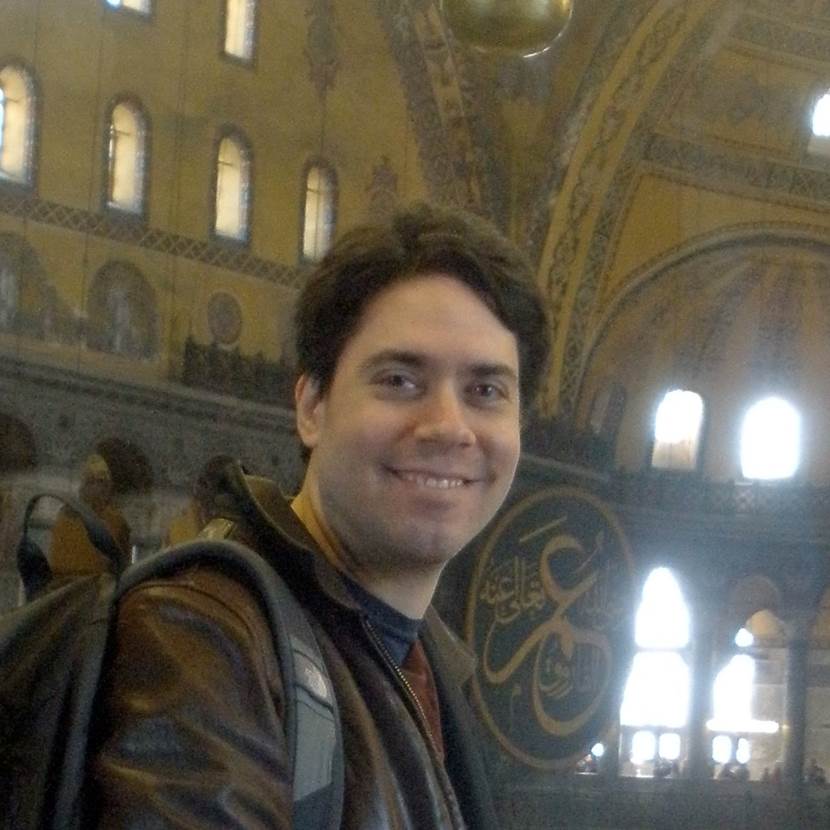