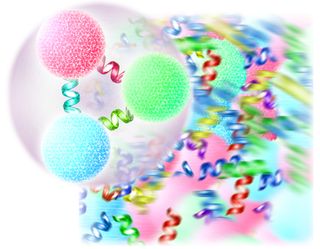
Hints of a mysterious particle that has been long suspected to exist but has never been spotted are being revealed in a new experiment.
So far, the elusive particles, called extra-heavy strange baryons, haven't been seen directly, but they are leaving tantalizing hints of their existence.
These extra-heavy strange baryons may be freezing out other subatomic particles in a plasma soup of subatomic particles that mimics conditions in the universe a few moments after the Big Bang, nearly 14 billion years ago. [5 Elusive Particles That May Lurk in the Universe]
Primordial soup
The particles were created during an experiment conducted inside the Relativistic Heavy Ion Collider (RHIC), an atom smasher at Brookhaven National Laboratory in Upton, New York. There, scientists created a soupy concoction of unbound quarks — the subatomic particles that make up protons and neutrons — and gluons, the tiny particles that bind quarks together and carry the strong nuclear force. Physicists think this quark-gluon plasma is similar to the primordial soup that emerged milliseconds after the universe was born.
Using the RHIC, physicists are trying to understand how quarks and gluons initially came together to form protons, neutrons and other particles that are categorized as hadrons. [Behind the Scenes at Humongous U.S. Atom Smasher]
"Baryons, which are hadrons made of three quarks, make up almost all the matter we see in the universe today," study co-author and Brookhaven theoretical physicist Swagato Mukherjee, said in a statement.
Sign up for the Live Science daily newsletter now
Get the world’s most fascinating discoveries delivered straight to your inbox.
Elusive matter
But while ordinary baryons are ubiquitous throughout the universe, the Standard Model — the physics theory that explains the bizarre world of subatomic particles — predicts the existence of a separate class of baryons made up of heavy or ''strange" quarks. These heavy baryons would exist only fleetingly, making them hard to spot.
If extra-heavy baryons did exist, they should leave some trace behind, scientists say.
Enter the RHIC experiment, which accelerates gold nuclei, or the protons and neutrons in a gold atom, to nearly the speed of light, and then crashes these gold ions into one another. The resulting collisions can raise the temperature inside the collider to a mind-boggling 7.2 trillion degrees Fahrenheit (4 trillion degrees Celsius), or 250,000 times as hot as the heart of the sun. The huge burst of energy released during the collision melts the protons and neutrons in the nuclei into their smaller components, quarks and gluons.
In this soupy plasma of quarks and gluons, Mukherjee and his colleagues noticed that other, more common, strange baryons were freezing out of the plasma at a lower temperature than would ordinarily be predicted. (There are several types of strange baryons.) The scientists hypothesized that this freezing-out occurred because the plasma contained as-yet-undiscovered hidden particles, such as hadrons composed of extra-heavy strange baryons.
"It's similar to the way table salt lowers the freezing point of liquid water," Mukherjee said in the statement. "These 'invisible' hadrons are like salt molecules floating around in the hot gas of hadrons, making other particles freeze out at a lower temperature than they would if the 'salt' wasn't there."
By combining their measurements with a mathematical model of quarks and gluons interacting in a 3D lattice, the team was able to show that extra-heavy strange baryons were the most plausible explanation for the RHIC's experimental results.
Now, the team is hoping to create a map of how different types of matter, such as quark-gluon plasma, change phases at different temperatures. Just as the chemical symbol H20 represents water in the form of a liquid, ice or steam depending on the temperature and pressure, the subatomic particles in an atom's nucleus take different forms at different temperatures. So, the team is hoping the new results could help them to create a map of how nuclear matter behaves at different temperatures.
The findings were reported Aug. 11 in the journal Physical Review Letters.
Follow Tia Ghose on Twitter and Google+. Follow Live Science @livescience, Facebook & Google+. Original article on Live Science.
Tia is the managing editor and was previously a senior writer for Live Science. Her work has appeared in Scientific American, Wired.com and other outlets. She holds a master's degree in bioengineering from the University of Washington, a graduate certificate in science writing from UC Santa Cruz and a bachelor's degree in mechanical engineering from the University of Texas at Austin. Tia was part of a team at the Milwaukee Journal Sentinel that published the Empty Cradles series on preterm births, which won multiple awards, including the 2012 Casey Medal for Meritorious Journalism.