Is there a limit to how much the coronavirus can mutate?
The possibilities are seemingly endless.
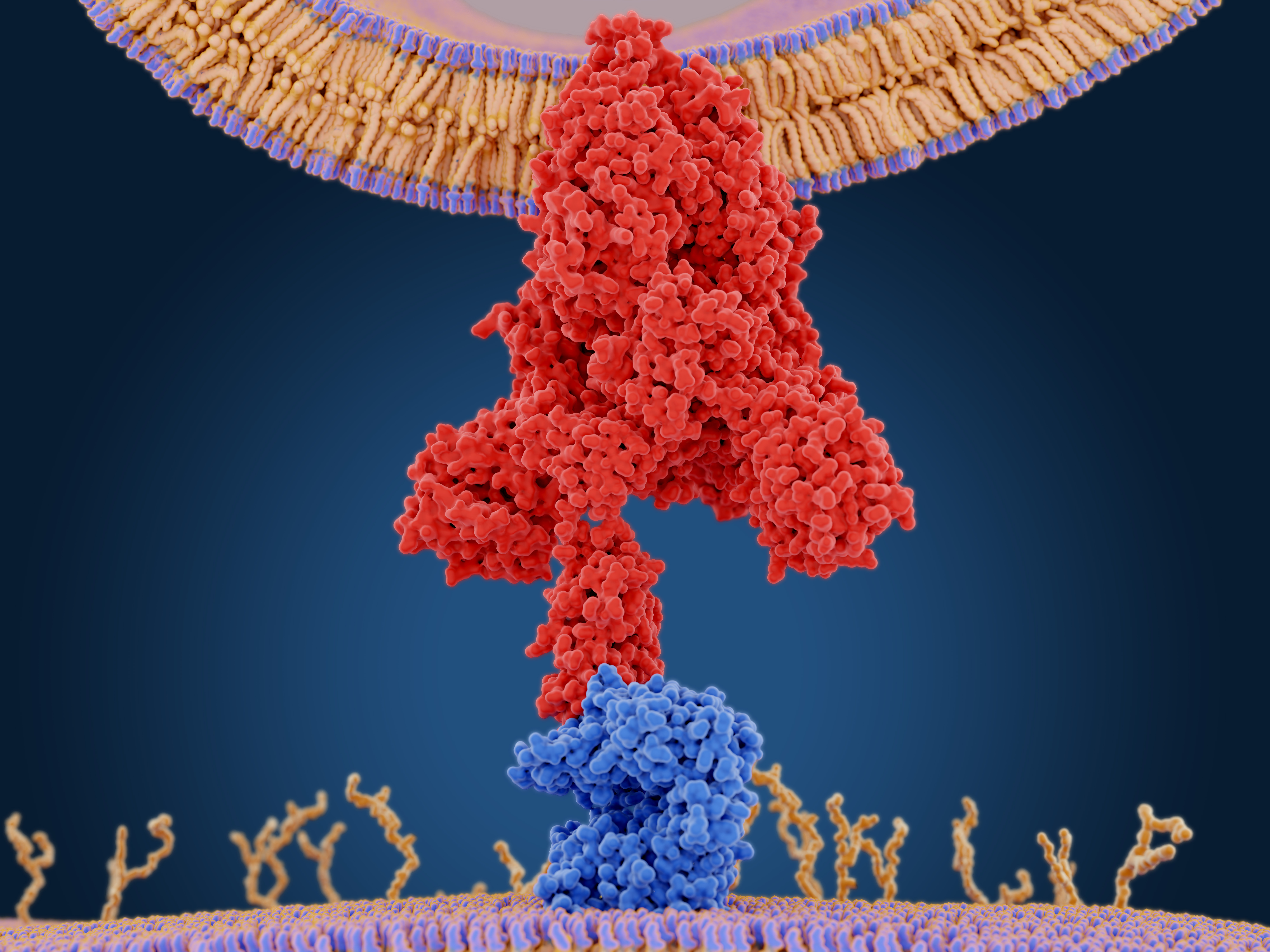
The coronavirus is mutating, picking up genetic changes as the world races to vaccinate people as fast as possible.
It's normal for viruses, including SARS-CoV-2, to mutate. But is there a limit to how much the virus can mutate and still make people sick — or can the virus just continue to evolve indefinitely?
It turns out there is a limit, but we don't exactly know what it is; and we can't begin to predict all of the possible mutations the virus could undergo, virologists told Live Science. The number of possible genetic mutations is greater than all the atoms in the visible universe, said Vincent Racaniello, a professor of microbiology and immunology at Columbia University in New York City. "A good fraction of the genome can be replaced."
Related: All your questions answered on UK coronavirus variant
The coronavirus's genetic code — made up of four different chemical bases or molecules that can be thought of as a four letter alphabet — is 29,881 letters long. Those letters provide instructions to make the 9,860 amino acids that are the building blocks of the virus's proteins. When those chemical bases change, amino acids also change, which can affect the shape of the virus’s proteins; those shape changes, in turn, can affect how the virus functions, such as how it binds to human cells.
Previous studies on other RNA viruses — which like SARS-CoV-2 have a single strand of RNA as their genetic material — have found that more than half of the bases in these viruses can be changed, Racaniello told Live Science. Mathematically, that means that if a virus is 10,000 base pairs long, there are 4^5000 genetic sequence possibilities.
That's incredibly big, considering there are 4^135 atoms in the visible universe. If the equation holds true for a virus like SARS-CoV-2, which has a genetic code three times as long, there could be 4^14,941 different possible combinations for its genetic sequence. And that's only counting basic letter changes — one chemical replacing another; there are also other mutations such as deletions or insertions in the genome that would further increase the number of possibilities, Racaniello said.
Sign up for the Live Science daily newsletter now
Get the world’s most fascinating discoveries delivered straight to your inbox.
But most of these mutations don't matter — and a huge fraction would be weeded out immediately. "Some mutations are lethal so we never see them," he said. And "many of them are neutral; they just accumulate and they're carried along." The mutations that matter are typically on the coronavirus's spike protein, the weapon it uses to latch onto the ACE2 receptors on the surfaces of human cells. The spike itself is made up of 1,273 amino acids, which are coded by 3,831 chemical bases; so by that same mathematical logic, there are 4^1916 ways the spike protein's code could vary, which is still nearly infinite. Still, many of these mutations are redundant and code for the same amino acids.
What's more, not every mutation will be identified as a new "variant," said John Moore, a professor of microbiology and immunology at Cornell University in Ithaca, New York. "Mutations that are silent happen, but they're not relevant." The variants that are identified and are given a name will usually have noteworthy properties — such as a greater ability to transmit to humans or to escape vaccines.
Robust surveillance can help scientists scan for changes in the virus's genome through random sampling of the infected population. Once they identify potentially important mutations — for example based on where the changes appear in the genome — they can plug those mutations into a computer model of the spike protein interacting with the ACE2 receptor to make predictions on how the variant will behave. But to ultimately understand how the mutation changed the behavior of the virus, they have to do experiments on the virus or proteins, Moore said.
Past mutations
The very first SARS-CoV-2 variant was identified last spring when the original virus first detected in Wuhan was replaced by a new variant called D614G, which likely emerged in Europe, according to a JAMA perspective that Moore co-authored. Not until late summer did scientists discover that the main mutation in that variant likely increased its ability to replicate and spread, Moore said.
While SARS-CoV-2 doesn't mutate as much as HIV or influenza does, "you put a virus like this in 100 million people and mutations are going to occur," Moore told Live Science. In August, another variant called B.1.1.7 emerged in the U.K., and its spread accelerated in November. Early studies suggest that the variant’s major mutation, called N501Y, again increased transmissibility, but likely didn't evade neutralizing antibodies that developed in response to D614G and other earlier strains of the novel coronavirus.
Related: All your questions answered on South Africa variant
Separately, a more worrying mutation took hold in South Africa. This one, called B.1.351 or N501Y.V2, has a similar mutation to the U.K. variant; but this one also has other mutations located close to the receptor binding site of the spike protein — the spot where the spike protein binds to ACE2 on human cells. The key mutation here is one called E484K, which changes the shape of the receptor binding domain (RBD) enough that antibodies that recognized earlier strains might have a hard time recognizing this new one.
Several early studies have suggested that vaccines, such as those by Moderna, Pfizer, Novavax and Johnson &Johnson, still protect against this variant but don't work as well against it as they do for the earlier variants, which they were designed to target.
Another similar variant to B.1.351, called P.1, also cropped up in Brazil, and because of its similarities to the South Africa variant, it could also be concerning. Now, scientists are finding a handful of B.1.1.7 variants that have also mutated to include the E484K mutation.
The RBD domain is one — but not the only — concerning spot where mutations can occur. It's made up of 223 amino acids, 22 of which make contact with the ACE2 receptor on human cells, Racaniello said. Any one of these amino acids can change (due to mutations in the domain’s underlying genetic sequence) and increase its contact with human cells, and thus its ability to invade.
Why are all these mutations happening?
Mutations sometimes reflect the so-called founder effect. The virus mutates and a variant becomes dominant because it happened to hop into a person who spread the virus widely. That doesn't necessarily mean that there's an advantage to the mutation.
Related: 20 of the worst epidemics and pandemics in history
But sometimes the same — or very similar— mutations, such as N501Y, pop up in different parts of the world. That normally means that the mutation confers an advantage to the virus, said Mohsan Saeed, an assistant professor of biochemistry at Boston University School of Medicine and an investigator in the university’s National Emerging Infectious Diseases Laboratories.
The virus is already extremely good at infecting people, so any future advantage it gains from mutations, will likely not be dramatic, he said. "If your radio is already very loud at volume 10, turning it up to 11 is not going to make a huge difference," Saeed told Live Science.
Still, "we will see what happens when the vaccine is administered at a massive scale," he said. In such situations, the vaccines may spur the viruses to develop "escape mutations" to block the ability of neutralizing antibodies.
Future mutations
SARS-CoV-2 has been circulating in the human population for only a short time, so "scientists are not yet in a position to make predictions about what variants will emerge in the future," based on simulations or past history with the virus, Saeed said. "It is mainly stochastic," or random, he said.
We're not calling the shots, we're reacting, Moore said. In other words, mutations are occurring in the wild and scientists are then figuring out what their impact will be on transmissibility, lethality or vaccine escape. Though it would be nice to be one step ahead of the virus — for instance predicting, based on simulations, what other mutations could emerge — that's likely not feasible given the extent to which the virus can mutate.
Related: Quick Guide on COVID-19 vaccines and how they work
"Proteins are very flexible in their abilities to interact with receptors or antibodies; they are capable of [tolerating] mutation in quite a number of different ways to achieve the same endpoint," Moore said. And so "you cannot predict what's going to happen."
Scientists may be able to predict some very obvious mutations that can pop up, such as certain amino acid changes on the spike protein that impact antibody binding or alterations in the spike's RBD which would impact the virus's ability to stick to and enter human cells, Racaniello said. "But that is only part of [the] spike and many other changes can impact fitness."
Though scientists can't predict which mutations will give the virus an edge, they know those mutations will emerge the more the virus spreads.
"You're going to see variants that are selected for greater transmissibility and/or antibody resistance because they're the two biggest selection pressures," Moore said.
Strong selection pressure, such as with very high efficacy vaccines, may reduce the number of chances for the virus to replicate and mutate. Meanwhile a really weak selection pressure means the virus doesn't have to mutate, so any change will provide a negligible advantage, Moore said.
The trouble comes when we put an intermediate level of selection pressure on the virus. For instance, widespread use of weak vaccines, or stretching out the time between the first and second doses of the vaccine, when you don't have a strong antibody response could be a "breeding ground for new variants," Moore said. "We're aware of that."
So to prevent future variants, we need to make sure we are giving people vaccines on schedule, to stop so-called escape mutants from emerging. And we need to stop the spread of the virus, which allows the coronavirus more chances to mutate.
These viruses "haven't suddenly grown scissors that will cut their way through masks, they haven't grown spring-heeled boots that will bounce them 50 yards between people," Moore said. "They're still coronaviruses and they're still stoppable by the standard procedures that we should all be doing."
Because we can't predict in advance which specific mutations will emerge. The only way to stay two steps behind the virus, rather than 20, is to dramatically ramp up surveillance for new variants, Moore said. That way, scientists can catch and test the impact of new variants in the lab before they become widespread.
If the variants become impervious to our vaccines, it's pretty simple to modify Pfizer's and Moderna's mRNA vaccines, to create booster shots by swapping out the genetic sequence used to teach the body to recognize the spike protein with the new mutated sequence, Live Science previously reported.
"The other point to remember is that when youre talking about these variants they haven't suddenly grown scissors that will cut their way through masks; they haven't grown spring heeled boots that will bounce them 50 yards between people," Moore said. "They're still coronaviruses and they're still stoppable by the standard procedures that we should all be doing."
Originally published on Live Science.
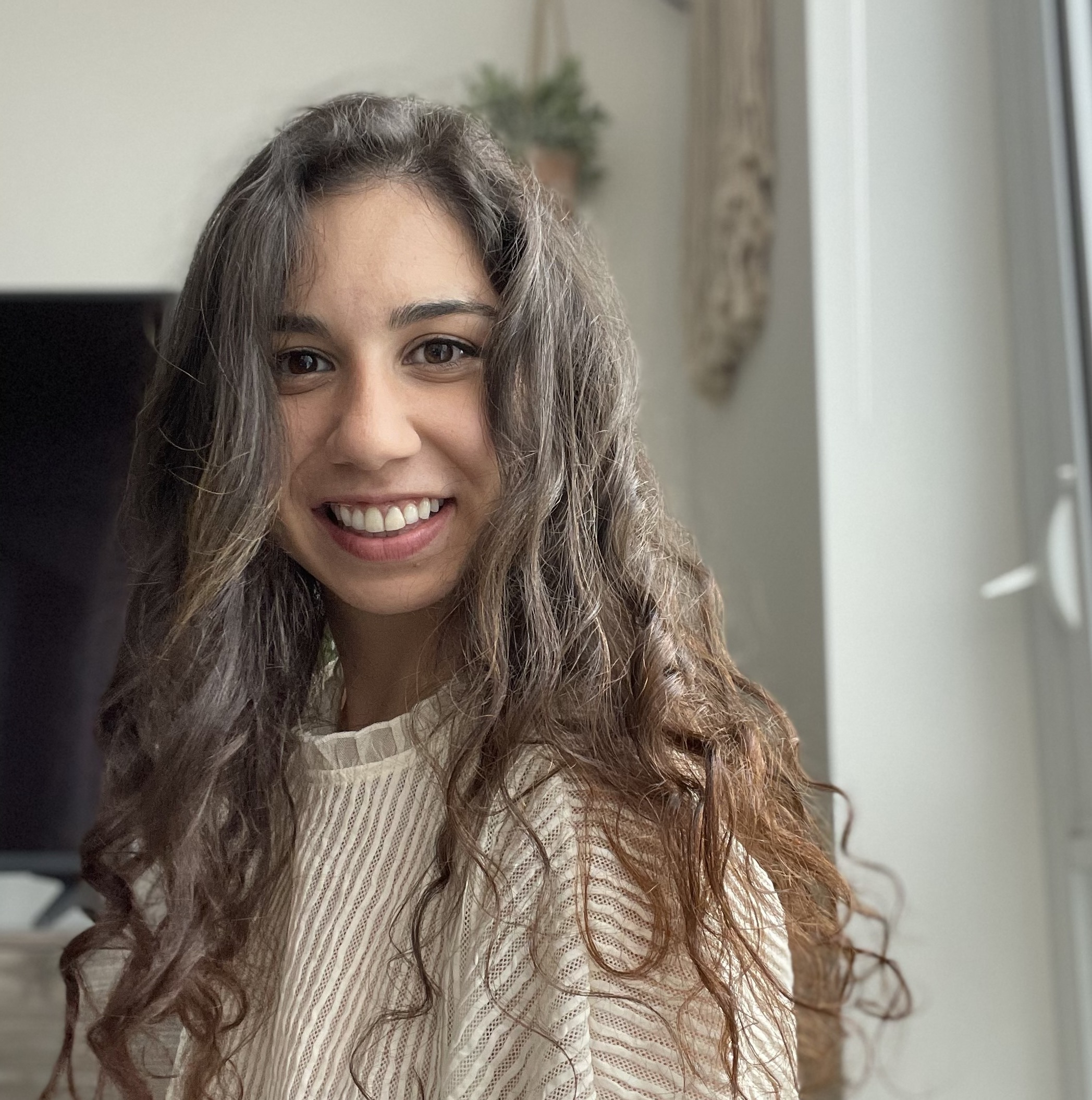
Yasemin is a staff writer at Live Science, covering health, neuroscience and biology. Her work has appeared in Scientific American, Science and the San Jose Mercury News. She has a bachelor's degree in biomedical engineering from the University of Connecticut and a graduate certificate in science communication from the University of California, Santa Cruz.