The Key to Life's Emergence? Bubbles, New Study Argues
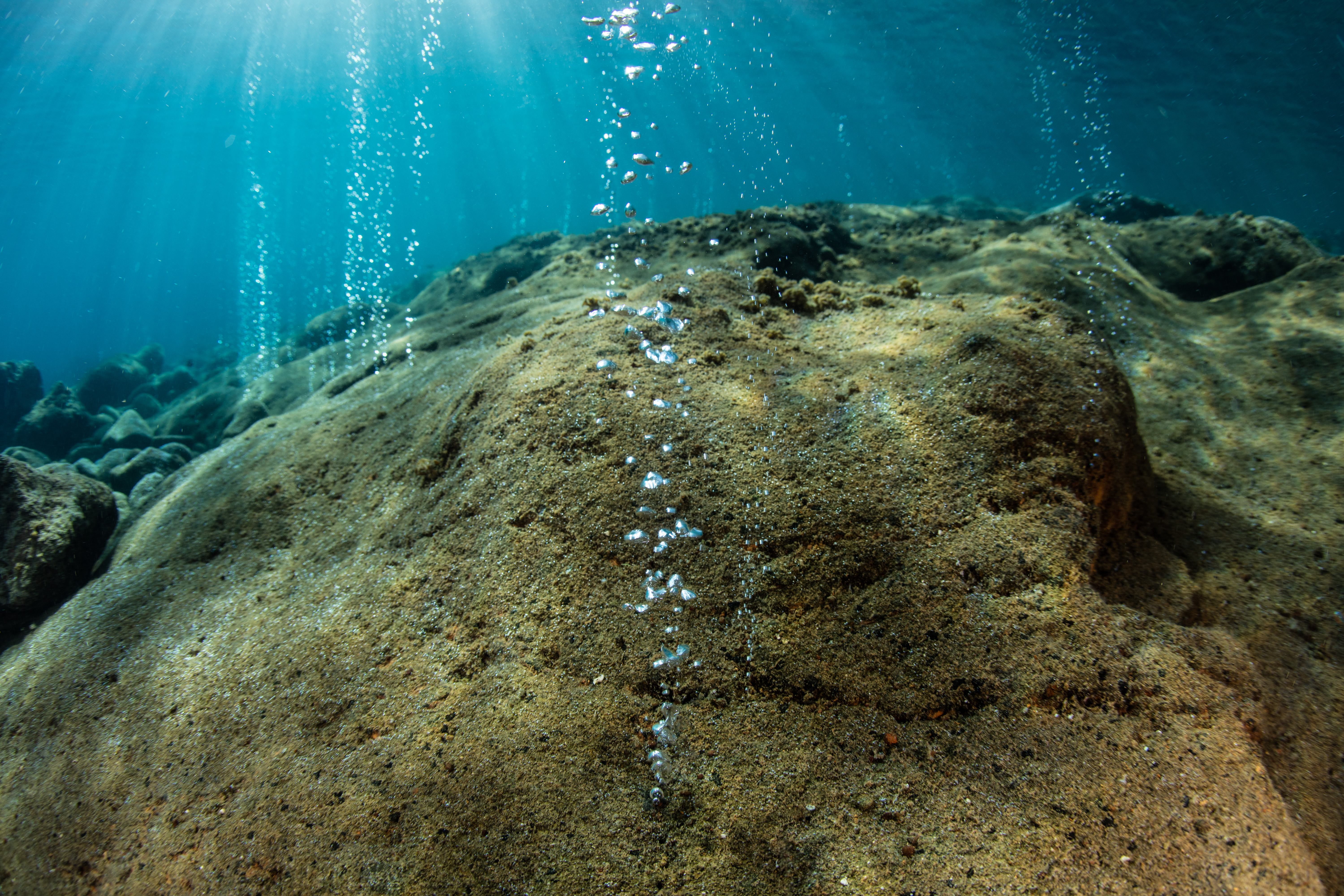
Before life on Earth emerged, by about 3.5 billion years ago, the oceans were a soup of randomly jumbled molecules. Then, somehow, some of those molecules arranged themselves into well-organized strings of DNA, protective cell walls, and tiny organ-like structures capable of keeping cells alive and functioning. But just how they accomplished this organization has long baffled scientists. Now, biophysicists at Ludwig–Maximilians University in Munich think they have an answer: bubbles.
The beginnings of life weren't instantaneous. Early precursor molecules somehow transformed into the building blocks of life, like RNA, DNA, salts and lipids. Then, those molecules organized to form the first early versions of cells, which then became the first single-celled organisms.
"This is the basis for all living species," Dieter Braun of Ludwig–Maximilians University, the lead author of the study, told Live Science.
In order for cells to form, begin replicating and take on a life of their own on primordial Earth, however, all the chemical parts first needed to come together, Braun said.
In the deep ocean, where many scientists think life got its beginnings, molecules like lipids, RNA and DNA may have been present; but even so, they would have been too spread out for anything interesting to happen.
"The molecules get lost. They diffuse," Braun said. "The reactions won't just happen by themselves."
Scientists agree that some force was necessary for the molecules to aggregate and react with one another, Henderson Cleaves, a chemist at Tokyo Institute of Technology, told Live Science. Researchers just don't agree what that force was.
Sign up for the Live Science daily newsletter now
Get the world’s most fascinating discoveries delivered straight to your inbox.
That's where bubbles come in.
Bubbles were everywhere in Earth's early seascape. Warm, deep-sea volcanoes spurted fizzy plumes. Those airy orbs, settled on the porous volcanic rock. These were the conditions that Braun and his colleagues sought to replicate. They created a vessel out of a porous material that mimicked the texture of volcanic rock, then filled it, in turn, with six different solutions, each modeling a different stage in the life-formation process. One solution, representing an early step, contained a sugar called RAO, which would have been necessary in the construction of nucleotides, the building blocks of RNA and DNA. Other solutions, representing the later stages, contained RNA itself, as well as the fats necessary to construct cell walls. [7 Theories on the Origin of Life]
Then, the researchers heated the solution on one end and cooled it on the other. They were creating something called a "thermal gradient," in which the temperature gradually changes from one end to another, similar to the way the water near deep-sea thermal vents gradually changes from hot to cold.
"It's like a micro-ocean," Braun said.
In each solution, the temperature change forces the molecules to clump — and they gravitated toward the bubbles that naturally form under these conditions. Almost immediately, they began reacting.
Sugars formed crystals, a kind of skeleton for RNA and DNA nucleotides. Acids formed longer chains, taking another step toward the formation of complex, RNA-like molecules. Finally, the molecules arranged themselves into structures that resembled simple cells. In a basic sense, Braun said, cells are molecules encased in bags made of fats. That's exactly what happened on the surface of his bubbles: Fats arranged themselves in spheres around the RNA and other molecules.
Most surprising to Braun and his colleagues, he said, was how rapidly these changes happened, in under 30 minutes.
"I was amazed," he said. Though this is the first time he and his colleagues have looked specifically at bubbles, the researchers have previously tried to replicate how these biological molecules undergo the complex reactions needed for life. Normally, he said, these reactions take hours.
Some chemists are skeptical, however, that Braun's bubbles are an accurate representation of the primordial environment. Braun and his colleagues seeded their solution with many of the complex molecules needed for life. Even their simplest solutions still represented later stages of the life-formation process, Ramanarayanan Krishnamurthy, a chemist at the Scripps Institution of Oceanography who was not involved in the study, told Live Science. That's a bit like baking a cake with a box mix, rather than starting from scratch.
In contrast, the ancient oceans may not have had the right conditions to form these initial molecules, Krishnamurthy said.
Plus, the bubble experiment took place on a tiny scale. That's important, because it means the change in temperature from one end of the test to the next was very abrupt. In reality, the thermal gradients under the ocean are more gradual, Cleaves said.
Still, Braun argued that there are a few reasons why bubbles might be the ideal place for the beginnings of life. First, they provide a perfect interface between air and water. Without air, many of the reactions necessary for life couldn't happen. For instance, phosphorylation, a reaction that enables small molecules to form complex molecular strings, has to happen under at least partially dry conditions. Inside the bubbles, that's not a problem; even though they're tiny, bubbles provide the perfect environment for these reactions to dry out, at least temporarily.
But there's another important role bubbles can play: They create order. In still water, molecules typically spread out with no particular arrangement. Bubbles, however, give molecules — and perhaps the beginnings of life — something to cling to in a chaotic world.
Originally published on Live Science.
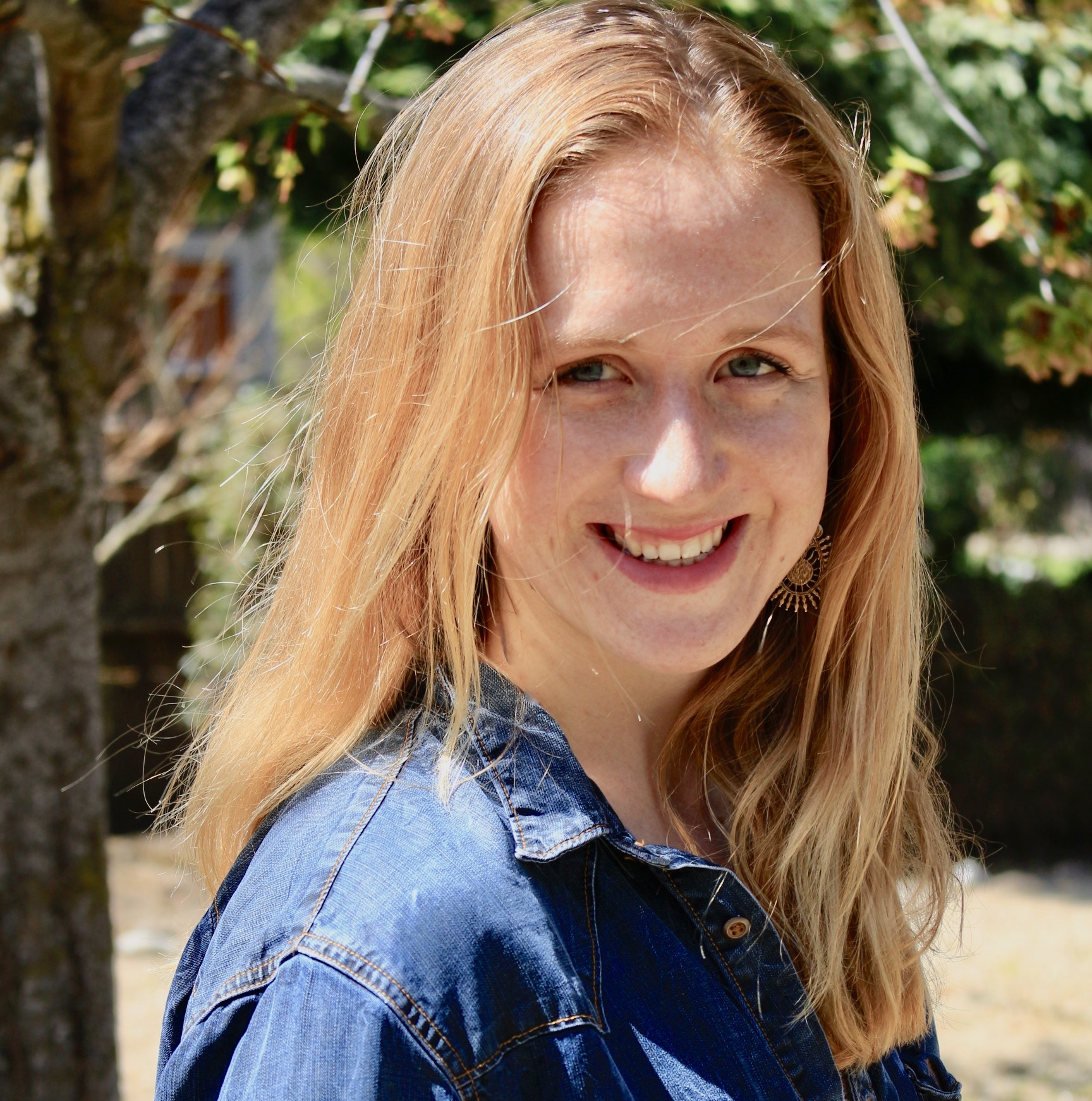
Isobel Whitcomb is a contributing writer for Live Science who covers the environment, animals and health. Her work has appeared in the New York Times, Fatherly, Atlas Obscura, Hakai Magazine and Scholastic's Science World Magazine. Isobel's roots are in science. She studied biology at Scripps College in Claremont, California, while working in two different labs and completing a fellowship at Crater Lake National Park. She completed her master's degree in journalism at NYU's Science, Health, and Environmental Reporting Program. She currently lives in Portland, Oregon.