Map Reveals Ghostly Antineutrinos Lurking Within Earth
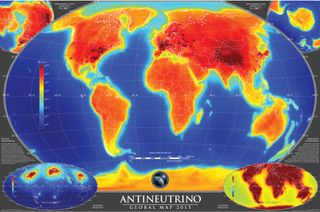
A look inside of Earth has revealed the hiding places of weird antimatter particles that are nearly massless, resulting in a global map of the planet's so-called antineutrinos.
Antineutrinos are the antimatter versions of neutrinos, particles so light and insubstantial that they rarely interact with matter. They can pass through a light-year of solid lead and still have a 50-50 chance of sailing through as if it wasn't there.
These subatomic particles are churned out as byproducts from nuclear reactions that fuel stars, the violent deaths of massive stars — called supernovas — black holes and nuclear reactors here on Earth.
The researchers of the new study focused on another producer of neutrinos and antineutrinos: the decay of radioactive elements deep within Earth. Heat from the radioactive decay, along with the heat left over from the formation of our planet, powers the movement of the planet's tectonic plates, a process that can trigger earthquakes and volcanic eruptions. [5 Mysterious Particles That May Lurk Beneath Earth's Surface]
As such, the new maps could help geologists figure out just how much radioactive material there is in the mantle as opposed to the crust, and from that, they can figure out how fast the Earth is cooling off from its fiery origins.
Seeing the invisible
The antineutrinos in the new study come from the radioactive decay of uranium and thorium. When a uranium or thorium nucleus splits in radioactive decay, it releases an antineutrino. The antineutrinos have a specific range of energies. Once in a while, one will smack into a proton, the nucleus of a hydrogen atom. When this happens, the proton is changed into a positron (an electron with a positive charge) and a neutron. The neutron, meanwhile, collides with another hydrogen atom and becomes deuterium — essentially, a heavier form of hydrogen.
Sign up for the Live Science daily newsletter now
Get the world’s most fascinating discoveries delivered straight to your inbox.
These neutron-hydrogen atom collisions create a double flash of light, the researchers said. These are hard to see, though, because they happen so rarely, since antineutrinos and neutrinos don't interact with matter that often — imagine firing a billion bullets at a target but only hitting it once. To catch the fleeting collisions requires detectors with lots of protons for the neutrinos to hit.
One of the underground detectors used in the study, the Kamioka Liquid Scintillator Antineutrino Detector (KamLAND) in Japan, for example, uses 1,000 tons of liquid (a mix of benzene and mineral oil, along with some fluorescent chemicals) in order to catch the few antineutrinos that will actually hit something.
The other is just as impressive: The Borexino detector, located about a mile (1.5 kilometers) under the Apennine Mountains in Italy, contains 300 tons of organic liquid for the antineutrinos to interact with, and more than 2,200 sensors to detect the resulting flashes of light. The entire detector, which is part of the Gran Sasso National Laboratory, is housed inside a huge sphere surrounded by water.
The researchers used data from both detectors to zero in on the antineutrinos being produced naturally deep inside Earth.
Missing heat
William McDonough, a co-author of the new study and a professor of geology at the University of Maryland, told Live Science that studying antineutrinos deep inside the planet can help scientists pin down models of the Earth's mantle.
"Ever since [the time of mathematician and physicist William Thomson], there's been debate about the heat budget of the Earth," McDonough said.
Current estimates of how much the Earth has cooled over each billion years since the planet formed can vary by 50 percent. These calculations are complicated because they have to account for the insulating effects of the Earth's crust, and make assumptions about concentrations of radioactive elements in the mantle, McDonough added.
That's where the new antineutrino map can come in handy. Such maps could offer insights into how much of the Earth's uranium and thorium ended up in the crust and how much ended up in the mantle.
What went where, and when
As antineutrino maps become more widespread and more precise, it should be possible to glean the bulk amounts of uranium and thorium in the Earth's mantle. For example, scientists know approximately how much uranium is in the planet's crust because the material can be mined from the ground to fuel nuclear power plants. But, estimates of the abundance of uranium and thorium in the mantle vary widely. One conservative estimate is approximately 10 parts per billion of uranium, McDonough said.
"That would mean about 70 percent of the uranium is in the crust," he said. At 30 parts per billion, three-fourths of Earth's uranium is in the mantle.
Studying these naturally occurring elements also has implications for understanding how planets form, McDonough said. But aside from revealing the structure of the Earth, such maps can help find illicit nuclear reactors around the world. A project in Ohio is being developed to use the detection of antineutrinos specifically for that purpose, and experiments like this one demonstrate it is possible to subtract background noise in the form of naturally occurring radioactive elements on Earth and from cosmic sources. In fact, future updates to this antineutrino map will show where existing nuclear reactors are decommissioned (or where new ones are started up), the researchers said.
A more complete map would tell a fuller story, but that would mean building more detectors. "One of the more fanciful ideas we've been trying to sell is to build a neutrino detector and drop it to the bottom of the Pacific Ocean," McDonough said. "You get a really nice mantle signal there."
The study was published Sept.1 in the journal Scientific Reports.
Follow Live Science @livescience, Facebook & Google+. Original article on Live Science.