What Are X-Rays?
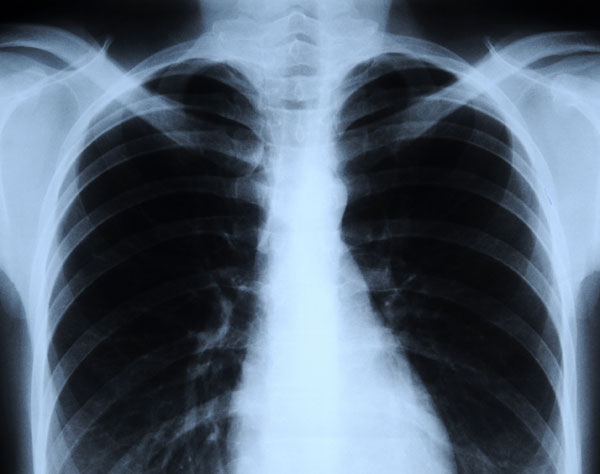
X-rays are types of electromagnetic radiation probably most well-known for their ability to see through a person's skin and reveal images of the bones beneath it. Advances in technology have led to more powerful and focused X-ray beams as well as ever greater applications of these light waves, from imaging teensy biological cells and structural components of materials like cement to killing cancer cells.
X-rays are roughly classified into soft X-rays and hard X-rays. Soft X-rays have relatively short wavelengths of about 10 nanometers (a nanometer is one-billionth of a meter), and so they fall in the range of the electromagnetic (EM) spectrum between ultraviolet (UV) light and gamma-rays. Hard X-rays have wavelengths of about 100 picometers (a picometer is one-trillionth of a meter). These electromagnetic waves occupy the same region of the EM spectrum as gamma-rays. The only difference between them is their source: X-rays are produced by accelerating electrons, whereas gamma-rays are produced by atomic nuclei in one of four nuclear reactions.
History of X-rays
X-rays were discovered in 1895 by Wilhelm Conrad Röentgen, a professor at Würzburg University in Germany. According to the Nondestructive Resource Center's "History of Radiography," Röentgen noticed crystals near a high-voltage cathode-ray tube exhibiting a fluorescent glow, even when he shielded them with dark paper. Some form of energy was being produced by the tube that was penetrating the paper and causing the crystals to glow. Röentgen called the unknown energy "X-radiation." Experiments showed that this radiation could penetrate soft tissues but not bone, and would produce shadow images on photographic plates.
For this discovery, Röentgen was awarded the very first Nobel Prize in physics, in 1901.
X-ray sources and effects
X-rays can be produced on Earth by sending a high-energy beam of electrons smashing into an atom like copper or gallium, according to Kelly Gaffney, director of the Stanford Synchrotron Radiation Lightsource. When the beam hits the atom, the electrons in the inner shell, called the s-shell, get jostled, and sometimes flung out of their orbit. Without that electron, or electrons, the atom becomes unstable, and so for the atom to "relax" or go back to equilibrium, Gaffney said, an electron in the so-called 1p shell drops in to fill the gap. The result? An X-ray gets released.
"The problem with that is the fluorescence [or X-ray light given off] goes in all directions," Gaffney told Live Science. "They aren't directional and not focusable. It's not a very easy way to make a high-energy, bright source of X-rays."
Enter a synchrotron, a type of particle accelerator that accelerates charged particles like electrons inside a closed, circular path. Basic physics suggests that any time you accelerate a charged particle, it gives off light. The type of light depends on the energy of the electrons (or other charged particles) and the magnetic field that pushes them around the circle, Gaffney said.
Since the synchrotron electrons are pushed to near the speed of light, they give off enormous amounts of energy, particularly X-ray energy. And not just any X-rays, but a very powerful beam of focused X-ray light.
Synchrotron radiation was seen for the first time at General Electric in the United States in 1947, according to the European Synchrotron Radiation Facility. This radiation was considered a nuisance because it caused the particles to lose energy, but it was later recognized in the 1960s as light with exceptional properties that overcame the shortcomings of X-ray tubes. One interesting feature of synchrotron radiation is that it is polarized; that is, the electric and magnetic fields of the photons all oscillate in the same direction, which can be either linear or circular.
"Because the electrons are relativistic [or moving at near light-speed], when they give off light, it ends up being focused in the forward direction," Gaffney said. "This means you get not just the right color of light X-rays and not just a lot of them because you have a lot of electrons stored, they're also preferentially emitted in the forward direction."
X-ray imaging
Due to their ability to penetrate certain materials, X-rays are used for several nondestructive evaluation and testing applications, particularly for identifying flaws or cracks in structural components. According to the NDT Resource Center, "Radiation is directed through a part and onto [a] film or other detector. The resulting shadowgraph shows the internal features" and whether the part is sound. This is the same technique used in doctors' and dentists' offices to create X-ray images of bones and teeth, respectively.[Images: Stunning Fish X-rays]
X-rays are also essential for transportation security inspections of cargo, luggage and passengers. Electronic imaging detectors allow for real-time visualization of the content of packages and other passenger items.
The original use of X-rays was for imaging bones, which were easily distinguishable from soft tissues on the film that was available at that time. However, more accurate focusing systems and more sensitive detection methods, such as improved photographic films and electronic imaging sensors, have made it possible to distinguish increasingly fine detail and subtle differences in tissue density, while using much lower exposure levels.
Additionally, computed tomography (CT) combines multiple X-ray images into a 3D model of a region of interest.
Similar to CT, synchrotron tomography can reveal three-dimensional images of interior structures of objects like engineering components, according to the Helmholtz Center for Materials and Energy.
X-ray therapy
Radiation therapy uses high-energy radiation to kill cancer cells by damaging their DNA. Since the treatment can also damage normal cells, the National Cancer Institute recommends that treatment be carefully planned to minimize side effects.
According to the U.S. Environmental Protection Agency, so-called ionizing radiation from X-rays zaps a focused area with enough energy to completely strip electrons from atoms and molecules, thus altering their properties. In sufficient doses, this can damage or destroy cells. While this cell damage can cause cancer, it can also be used to fight it. By directing X-rays at cancerous tumors, it can demolish those abnormal cells.
X-ray astronomy
According to Robert Patterson, professor of astronomy at Missouri State University, celestial sources of X-rays include close binary systems containing black holes or neutron stars. In these systems, the more massive and compact stellar remnant can strip material from its companion star to form a disk of extremely hot X-ray-emitting gas as it spirals inward. Additionally, supermassive black holes at the centers of spiral galaxies can emit X-rays as they absorb stars and gas clouds that fall within their gravitational reach.
X-ray telescopes use low-angle reflections to focus these high-energy photons (light) that would otherwise pass through normal telescope mirrors. Because Earth's atmosphere blocks most X-rays, observations are typically conducted using high-altitude balloons or orbiting telescopes.
Additional resources
- To learn more, download this PDF from SLAC titled "Early History of X-Rays."
- The NDE/NDT Resource Center provides information about nondestructive evaluation/nondestructive testing.
- NASA's mission page on the electromagnetic spectrum explains how astronomers use X-rays.
This page was updated on Oct. 5, 2018 by Live Science Managing Editor, Jeanna Bryner.
Sign up for the Live Science daily newsletter now
Get the world’s most fascinating discoveries delivered straight to your inbox.
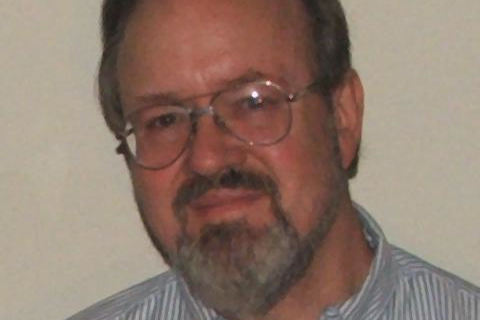