
Neutrons' 'evil twins' may be crushing stars into black holes
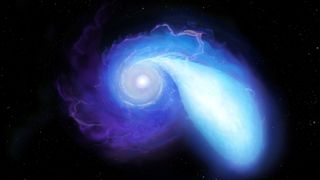
The universe may be filled with "mirror" particles — and these otherwise-undetectable particles could be shrinking the densest stars in the universe, turning them into black holes, a new study suggests.
These hypothetical evil twins of ordinary particles would experience a flipped version of the laws of physics, as if the rules that govern known particles were reflected in a looking glass. According to a new study, published in December 2020 in the preprint database arXiv but not yet peer-reviewed, if these particles exist, they would be shrinking the densest stars in the universe into black holes.
Related: 10 huge black hole findings
Through the looking glass
Several fundamental symmetries in nature give rise to the laws of physics. For example, the ability to move an experiment or interaction in space and have the same outcome leads to conservation of momentum.
But one of these symmetries, the symmetry of reflection, isn't always obeyed.The symmetry of reflection is when you look at the mirror image of a physical reaction. In almost all cases, you get the exact same result. For example, if you toss a ball up in the air and catch it, it looks exactly the same in the mirror — the gravity respects reflection symmetry.
But not all forces play along. The violator of reflection symmetry (also known as mirror symmetry, P-symmetry or parity) is the weak nuclear force. Whenever the weak force is involved in some particle interaction, the mirror image of that interaction will look different. The classic experiment that first detected this effect found that when a radioactive version of cobalt decays, the electron it emits prefers to go in one direction (in particular, opposite the direction of the spin of the cobalt), rather than any random direction. If the weak nuclear force obeyed reflection symmetry, then those electrons shouldn't have "known" which direction is which, and popped out anywhere they please.
Physicists have no idea why the mirror symmetry is broken in our universe, so some have proposed a radical explanation: Maybe it's not broken at all, and we're just looking at the universe the wrong way.
Sign up for the Live Science daily newsletter now
Get the world’s most fascinating discoveries delivered straight to your inbox.
You can rescue mirror symmetry if you allow for the existence of some extra particles. And by "some," I mean "a lot" — a mirror-image copy of every single particle. There would be mirror electrons, mirror neutrons, mirror photons, mirror Z bosons. You name it, it's got a mirror. (This is different from antimatter, which is like normal matter but with opposite electric charge.)
Other names for mirror matter include "shadow matter" and "Alice matter" (as in, "Through the Looking-Glass"). By introducing mirror matter, reflection is preserved in the universe: Ordinary matter performs left-handed interactions, and mirror matter performs right-handed interactions. Everything syncs up at the mathematical level.
The heart of the star
But how can scientists test this radical idea? Because the only force that violates mirror symmetry is the weak nuclear force, that's the only force that can provide a "channel" for regular matter to communicate with its mirror counterparts. And the weak force is really, really weak, so even if the universe were flooded with mirror particles, they'd be barely detectable.
Many experiments have focused on neutral particles, like neutrons, because they don't have electromagnetic interactions, thereby making the experiments easier. Searches for mirror neutrons haven't turned up anything yet, but all hope is not lost. That's because those experiments have taken place on Earth, which doesn't have a superstrong gravitational field. But theoretical physicists predict a very strong gravitational field can enhance the connection between neutrons and mirror neutrons. Thankfully, nature has already crafted a far superior experimental device to hunt for mirror matter: neutron stars.
Neutron stars are the leftover cores of giant stars. They are extraordinarily dense — a single teaspoon of neutron star material would outweigh the Great Pyramids — and extremely small. Imagine cramming 10 suns' worth of material into a volume no bigger than Manhattan.
Neutron stars are essentially city-size atomic nuclei composed of individual neutrons crammed together just about as tightly as possible.
Neutrons' evil twins
With that incredible neutron abundance, coupled with the extreme gravitational field (the tallest "mountains" on neutron stars are barely a half inch high), weird things are bound to happen. One of those things, the new study proposes, is neutrons occasionally turning into their mirror neutron counterparts.
When a neutron turns into a mirror neutron, a few things happen. The mirror neutron still hangs out inside the star; it's gravitationally bound and thus can't go anywhere. And the mirror neutron has a (tiny) gravitational influence of its own, so the star doesn't evaporate. But mirror neutrons don't participate in the interactions that scientists detect in neutron stars, so that changes the internal chemistry. They do take part in a "mirror neutron star" life, with its own set of interesting atomic interactions, but that life is hidden from us, like a ghost inhabiting the body of a regular neutron star.
It's like going to a crowded football game and slowly replacing the fans with cardboard cutouts: The stadium is still filled, but the energy is gone.
As neutrons slowly convert to mirror neutrons, the star shrinks. At a 1:1 ratio of regular neutrons to mirror neutrons, the neutron star finds itself about 30% smaller.
Neutron stars can hold themselves up from the crushing weight of their own gravity by a quantum mechanical process called degeneracy pressure. But that pressure has a limit, and with fewer regular neutrons, that limit shrinks. If a star had a 1:1 ratio of ordinary neutrons to mirror neutrons, the maximum mass of neutron stars in the universe would be about 30% less massive than what we would normally expect. More massive than this, and neutron stars would collapse into black holes.
Scientists have observed neutron stars bigger than this, which at first glance might mean that mirror matter is a dead-end idea (and we have to find some other explanation for mirror symmetry violation). But the case isn't closed: The universe is only so old (13.8 billion years), and we have no idea how long this changeover process can take. It's possible there just hasn't been enough time for the neutron stars to make the switch.
The cool thing about neutron stars is that scientists are looking at them all the time. By finding and observing more neutron stars, they might just find a sign in any of those signals that there's a hidden, mirror — and dare I say "evil"? — sector of the universe.
Originally published on Live Science.
Paul M. Sutter is a research professor in astrophysics at SUNY Stony Brook University and the Flatiron Institute in New York City. He regularly appears on TV and podcasts, including "Ask a Spaceman." He is the author of two books, "Your Place in the Universe" and "How to Die in Space," and is a regular contributor to Space.com, Live Science, and more. Paul received his PhD in Physics from the University of Illinois at Urbana-Champaign in 2011, and spent three years at the Paris Institute of Astrophysics, followed by a research fellowship in Trieste, Italy.