Physicists trap ultracold plasma in a magnetic bottle for the 1st time
Scientists want to use it to learn more about the sun and nuclear fusion.
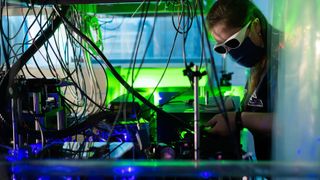
Capturing lightning in a bottle is the very definition of a tough task, but now physicists have found a way to contain ultracold plasma in a magnetic bottle trap, a breakthrough that could bring physicists one step closer to understanding solar winds and achieving nuclear fusion.
Plasma is one of the four states of matter, consisting of positive ions and negative free electrons. But unlike solids, liquids and gases, its tendency to occur in only the most extreme places, such as in the streak of ionized air we call a lightning bolt, in the dancing pattern of the aurora borealis, or on the surface of the sun, makes it extremely difficult to study.
This difficulty is only worsened by the fact that the plasmas in the Northern Lights or on the sun's surface interact with a complex magnetic field in ways scientists have yet to fully understand.
Related: 9 cool facts about magnets
"Throughout the sun's atmosphere, the (strong) magnetic field has the effect of altering everything relative to what you would expect without a magnetic field, but in very subtle and complicated ways that can really trip you up if you don't have a really good understanding of it," study co-author Peter Bradshaw, an astrophysicist at Rice University in Houston, said in a statement.
Colder particles move slower, allowing much more precise measurements of their behavior. In order to figure out how plasmas interact with magnetic fields, the scientists cooled their plasma, made of strontium, down to about 1 degree above absolute zero (around minus 272 degrees Celsius) using a technique called laser-cooling.
You'd think that firing a laser at something would heat it up, but if the photons (light particles) in the laser beam are traveling in the opposite direction of the moving plasma particles, they can actually cause those plasma particles to slow and cool them down.
Sign up for the Live Science daily newsletter now
Get the world’s most fascinating discoveries delivered straight to your inbox.
Once the plasma was cooled, the researchers trapped it momentarily with forces from surrounding magnets, allowing them to study it before it dissipated. They then set out to disentangle the interaction between the ions and electrons of the plasma and the magnetic field, which varies greatly across the plasma. The interaction was so complex that it took them a year to fully interpret their data.
"We measure plasma properties by scattering light off the ions in the plasma, but the magnetic field really complicates that," Rice Dean of Natural Sciences and corresponding author Tom Killian told Live Science. This is because the magnetic field changes how the ions scatter the laser light in very unpredictable ways.
"On top of that, the magnetic field is varying in space all across the plasma,” said Killian. “We had to sort out all of those effects." to paint a picture of the plasma density and speed across the bottle over time.
The picture they revealed was one where the fast-moving, low-mass electrons were tightly pinned to the magnetic field lines and spiraling around them, with the positive ions held inside the trap by their attraction to the negatively charged electrons. The paper's authors speculate that the magnetic field kept the electrons and ions from combining to form neutral atoms, and so kept the soup trapped in its plasma state.
The trapping technique opens up a wide range of avenues for plasma research. If physicists can capture ultra-cold plasma in a bottle, they can study the behavior of plasma-composed stellar objects like white dwarfs, or begin to replicate the conditions for fusion inside the sun.
Next, the researchers said they will design a laser grid that will plug any holes in the bottle's magnetic field through which ions could escape the experiment. They also hope to further investigate the processes that occur inside the trapped plasma, such as how the ions and electrons could recombine or how energy and mass move through the system.
"Our new abilities may give a great opportunity to study those phenomena," Killian said. "Similar effects are probably important for understanding some other systems that are hard to do experiments on, like white dwarf stars."
Originally published on Live Science.
Ben Turner is a U.K. based staff writer at Live Science. He covers physics and astronomy, among other topics like tech and climate change. He graduated from University College London with a degree in particle physics before training as a journalist. When he's not writing, Ben enjoys reading literature, playing the guitar and embarrassing himself with chess.