
Polar Ice May Hold Secrets of Futuristic Materials (Video)
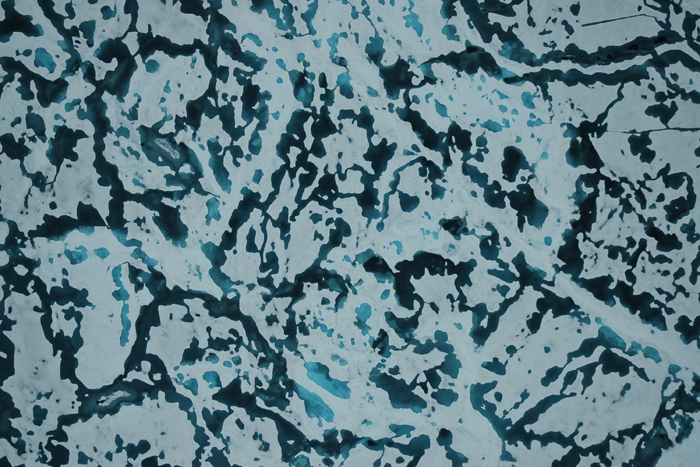
Amina Khan is a multimedia producer for the U.S. National Science Foundation (NSF). She contributed this article to Live Science's Expert Voices: Op-Ed & Insights.
What piques a penguin's curiosity? For a few curious birds, it's been mathematician Ken Golden drilling cores from Antarctic sea ice. Golden has been on 17 expeditions to the Arctic and Antarctic over the course of his career, teasing out the ice's unique structures. Understanding the structure of sea ice can reveal not only how fast sea ice will melt and the climate impact from shrinking polar ice caps , but also provide clues for better understanding composite materials — such as human bone, or polycrystalline media like rocks and metals — based on how ice crystals and brine intertwine in the microstructure of sea ice.
"Sea ice is a very complicated system," said Golden, who has been studying it firsthand since his first expedition to Antarctica, in 1980. "When you go down there," he said, "you see how it interacts with the ocean, how it interacts with the waves, with the atmosphere."
Ice, laced with brine
The interactions between the sea ice and its environment dramatically change the ice and how it behaves. That's mainly because sea ice, although it appears to be just solid ice, is actually a composite material, simply meaning it's made up of more than one ingredient.
Sea ice mostly forms two distinct structures: Columnar sea ice, more common in the Arctic, is made up of vertically oriented crystals jammed into extremely close quarters, with tiny pockets of brine filling out the submillimeter nooks and crannies between the pure ice platelets that conglomerate to make up each columnar crystal.
The second type, granular sea ice, is more commonly found in the Antarctic and is finer and more grainlike in its polycrystalline structure. Although both types of sea ice are made up of ice crystals and brine, their microstructure and fluid-flow properties differ substantially. And Golden is keenly interested in researching and describing those properties through his mathematics.
Stealth insights
With support from NSF, Golden has been studying composite materials and sea ice structure since 1984, when he was an NSF Mathematical Sciences Postdoctoral Fellow in mathematical physics at Rutgers University in New Jersey. Early in his work, he noticed how strikingly similar the porous microstructure of sea ice is to other composite materials, such as stealth radar-absorbing materials.
"It reminded me of what are called compressed-powder microstructures, where you have big polymer spheres and little metal particles, and then those microstructures are compressed down to form a matrix of an insulating polymer host with conducting inclusions," he said. In those microstructures, the conducting particles form interconnected electrical pathways around the insulating polymer particles, just as microscopic brine inclusions in sea ice may connect to form briny pathways around solid ice crystals.
Golden used math already devised to describe the critical volume threshold, or the volume percentage, of conducting particles required to obtain long electrical pathways and stealth capabilities in compressed powders. He then applied the compressed-powder model to the microstructural and fluid-flow properties of sea ice.
From this, he developed his Rule of Fives, which describes the conditions required for microscopic brine pathways to connect up and form larger-scale channels, thus allowing fluid flow in columnar sea ice. [See Golden discuss his Rule of Fives and his expeditions in this video]
It's cross-pollination, he said, between apparently disparate fields in science and engineering — structures that appear similar may also share the same underlying mathematics. But just as his mathematics applies ideas from other areas of science to sea ice research, the ideas he and his team develop for sea ice can also be applied to similar composite materials in other fields.
"For example," he said, "how to monitor osteoporosis in human bone, which turns out to be extremely close in structure to sea ice." That's because bone is a composite material, and the mathematics developed through sea ice research, specifically on the changes in the microstructure of sea ice over time, can also be used to describe changes in bone density and structure.
"And what I've studied as a mathematician is the effective properties of composite materials," said Golden. "Their effective electrical properties, their effective fluid-transport properties, their effective strength properties and so on." All of these can be applied to similar structures being studied or developed in fields of science and engineering apart from sea ice research. [Doing the Math on Polar Sea Ice Melt ]
Answers flow to the surface
But ask Golden to reduce his fascination with sea ice to its bare bones, and he'll sum it up in one word: percolation. That is the movement of water up and down through the complex microstructure of the ice. Percolation is one of the mechanisms important to both sea ice growth and melting, and is therefore essential for both the health and demise of the ice pack.
Sea ice often starts small when water freezes into tiny ice crystals floating near the ocean surface. As the ice layer thickens, larger crystals grow downward, where competing ice crystals bumping into each other may fuse, or one may win out, with the conglomerate eventually accreting into sea ice. This ice may gain thickness as water underneath it continues to freeze downward. It also grows through the accumulation of "snow ice," or ice formed from a slushy mix of precipitation and seawater, on the surface.
In a paper published in the Journal of Geophysical Research in 2008, Ted Maksym — then at the British Antarctic Survey — and Thorsten Markus of the NASA Goddard Space Flight Center found that depending on the season, snow-ice may account for anywhere from 23 to 43 percent of the total ice thickness. Snowfall is necessary to form the vast areas of slush covering Antarctic sea ice in winter, but where was the brine component of the snow-ice coming from?
Lateral intrusion of seawater on the surface, where the snow weighs the ice down below water level, flooding the ice from the sides, would account for some surface flooding. But much of the briny seawater, Golden said, may be coming up to the surface through the porous microstructure of the underlying sea ice, mixing with the surface precipitation and freezing into snow ice.
Golden witnessed this process himself on July 24, 1994, during the NSF-funded Antarctic Zone Flux Experiment (ANZFLUX) expedition to the eastern Weddell Sea. He witnessed water flooding the surface of the ice at the base of the snow layer during a snowstorm, which was warming the upper layers of the ice, making them permeable to upward brine percolation.
Sea ice as habitat
Percolation also brings up vital nutrients from the ocean, helping sustain algae that live within the brine inclusions. And although it sounds like these algae are isolated inside the ice from the larger marine ecosystem, that isn't the case.
On the sea ice off Barrow, Alaska, Columbia University marine ecologist Craig Aumack, another NSF-funded scientist, is researching how algae living in the sea ice tie into the surrounding marine ecosystem. He said algae start to bloom when temperatures warm up in spring.
"Then, as the snow completely melts," he adds, "they start getting lots and lots of light, [and] they migrate down to the bottom of the ice, and then eventually leave the ice [and enter] into the water column." That's where they become food for algae eaters that, in turn, contribute to the larger food cycle in the ocean.
Ice-dwelling algae have carved out a precarious existence inside the sea ice, relying, Golden said, on percolation for nutrients that come up from the ocean through the porous microstructure of sea ice. But whether fluid percolates through the ice depends on a delicate balance of temperature and salinity within the ice itself. Tilt the balance this way or that, and percolation does not occur.
Of ice and electromagnetism
Percolation occurs when the ice becomes permeable enough, but the conditions at which columnar sea ice becomes permeable differ from those for granular sea ice. That's because the way microscopic brine pockets are distributed in columnar sea ice is different from how they are arranged in granular sea ice. Zooming in even further into the ice microstructure, columnar and granular sea ice have different polycrystalline structures, which means their electromagnetic properties also differ.
In recent work featured on the cover of the February 8, 2015, issue of the Proceedings of the Royal Society of London A, Golden and his colleagues adapted a sophisticated mathematical theory into a new method for analyzing polycrystalline materials such as rocks, ceramics, metals and ice. They obtained rigorous mathematical relations between the electromagnetic properties of different polycrystalline structures and statistical data on how these structures' crystals are oriented — the direction in which they point, for instance. The results could then be used, for example, to distinguish between columnar and granular sea ice using just bulk electromagnetic data.
Although much of this work is mostly mathematical in nature, it has already been applied to sea ice, and could ultimately be used in the industrial manufacture of tailored polycrystalline structures. Materials of specific weight, tensile strength, electromagnetic, or thermal properties could potentially be made to order for applications ranging from aeronautics to construction, just by tailoring the orientations of the crystals in the manufacturing process.
The dangers of ice — beyond slipping
It's not just algae that live precariously. Golden has seen his share of near disasters.
In 1998, he was on the Australian icebreaker Aurora Australis, about 12 hours inside the ice edge in Antarctica, when the fire alarm went off. It wasn't a drill. A fire was raging in the engine room, the flames out of control. The nearest help was perhaps days away, with nothing for miles but water and ice.
Everyone was called to muster on the stern, the ship's first mate urging calm. The crew began preparing to lower the lifeboats, getting ready for the worst.
In the icy, vast waters near Earth's southern pole, fire can be as disastrous as it is terrifying: fiery flames on the one hand and on the other, a risky evacuation in waters too cold for human survival.
"And at that point," Golden recalled, "I'm getting particularly concerned. All of us are. Because the crew — these were very professional, extremely talented people — were very calm under trying conditions. But you could sense in their voice that this was a very, very serious situation."
How far is the nearest ship? How quickly would it be able to rescue the crew and passengers of the Aurora Australis if they all had to abandon ship — inside the sea ice pack? So many unknowns, each underlining the unasked question: Would they make it?
"You're down there," Golden said, "and you're on your own."
And any emergency decisions made could cost tremendously, in property or in human lives. The crew fighting the fire got out of the engine room just before an explosion ripped through it. As a last-ditch effort to save the ship, the captain deployed Halon gas — toxic to humans — to smother the flames. And it worked.
Luck was on their side, and no lives were lost that day. Everyone escaped with nothing worse than a grand scare.
In the warmth and safety of his office at the University of Utah, Golden dwells comfortably on that terrifying experience.
"We were basically there for five days. First two days with no power, no toilets or anything like that. So that was certainly a pretty harrowing experience, being in that situation."
It's this spirit of exploration in the pursuit of scientific knowledge that Golden said he hopes to pass on through his work. At the university, he works with students who want to make their own tracks in polar ice. Many do follow Golden to the Earth's poles for fieldwork in their areas of study.
Watching Golden demonstrate a meter-long ice-core drill to a couple of students, it is easy to see that he is as much at home here as he is on the ice, inspiring students from a range of disciplines: mechanical engineering, bioengineering, electrical engineering, physics and chemistry, to name just a few.
"I think, ultimately, what we'd really love to do is to bring more people into mathematics and show how mathematics is really the operating system of science and engineering," Golden said.
"Taking into account the role that mathematics plays as a universal language unifying natural sciences, it's not surprising that mathematics and its applications play so important and fundamental a role in so many disciplines," said program director Victor Roytburd of NSF’s Division of Mathematical Sciences. "Ken Golden's work is a textbook example of the application of mathematics to understanding complex natural phenomena. In a typical case, although the basic physical laws that govern elementary events in the growth and decay of sea ice are pretty clear, understanding the intricate interactions of such events on many scales requires an understanding of how these play together simultaneously. Golden's work contributes invaluable insights to understanding and perhaps managing the life and evolution of sea ice.”
What does this quest to understand sea ice mean for curious penguins in the Antarctic? They might soon find themselves rubbing wings with just that many more researchers chipping away at sea ice under the light of the polar sun. Here, the mathematics of the nature and behavior of composite structures and materials lie just beneath the surface.
Follow all of the Expert Voices issues and debates — and become part of the discussion — on Facebook, Twitter and Google+. The views expressed are those of the author and do not necessarily reflect the views of the publisher. This version of the article was originally published on Live Science.
Sign up for the Live Science daily newsletter now
Get the world’s most fascinating discoveries delivered straight to your inbox.