Brain Cells Viewed 'Talking' at Molecular Level
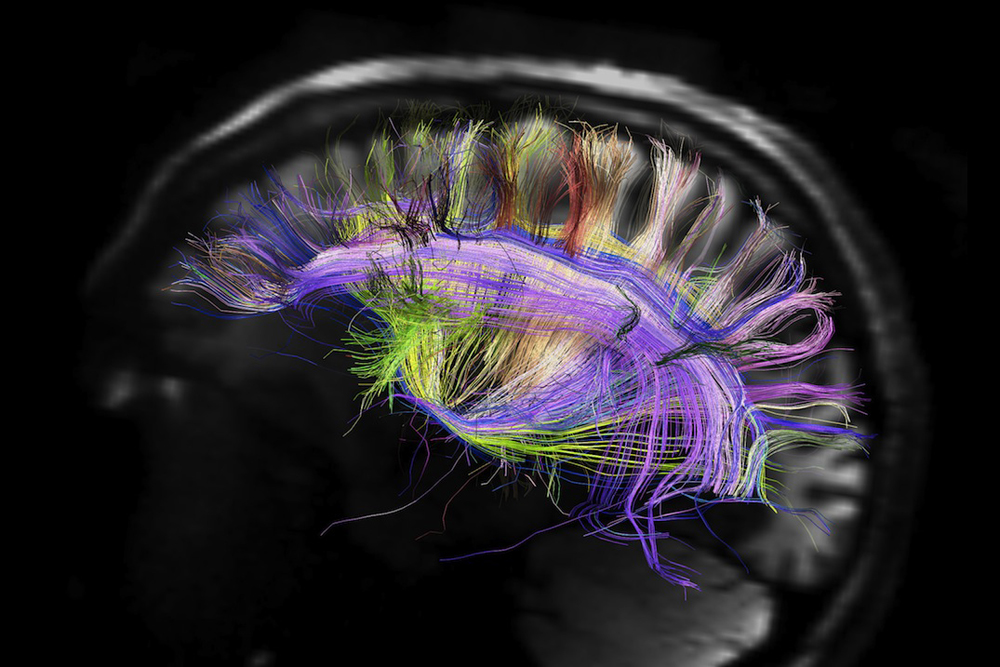
Brain scans are now starting to peer down to the molecular level, revealing what brain cells are telling one another, researchers say.
This new technique could illuminate the behavior of the human brain at its most fundamental level, yielding insights on disorders such as addiction, the scientists added. Right now the technique has been tested only on rats.
"This demonstrates a new way to study the brain — no one has ever mapped brain activity in this way before," said study author Alan Jasanoff, a bioengineer and neuroscientist at MIT.
One of the key ways researchers use to scan brains is magnetic resonance imaging, or MRI. These scanners immerse people in strong magnetic fields and then hit them with radio waves, encouraging atoms — usually hydrogen atoms — to emit signals that yield insights on the body.
By using MRIs to look at the hydrogen atoms in water, scientists can follow the flow of blood in the brain, shedding light on brain activity. However, this strategy, known as functional MRI, or fMRI, essentially reveals only what parts of the brain are talking, not what different areas of the brain are saying to each other. [See 3D Images of the Human Brain]
Now scientists are using novel molecules that can help them use fMRI to see what specific messages brain cells are sending each other.
The molecules they developed are based on a protein known as BM3h. "It's a heme protein, a type of protein that has an iron atom incorporated into its chemical structure, much like how the oxygen-carrying protein in your blood hemoglobin does," Jasanoff told Live Science. "The iron heart of BM3h is magnetically active, and can perturb the signal that water gives off during fMRI."
Sign up for the Live Science daily newsletter now
Get the world’s most fascinating discoveries delivered straight to your inbox.
In past work, the researchers altered BM3h to make it capable of binding onto neurotransmitters, the chemicals that brain cells use to send messages to each other. When these proteins bind onto their targets, "you will then see a little dip in the fMRI signal," Jasanoff said.
One of the modified proteins, known as BM3h-9D7, latches onto the neurotransmitter dopamine, which plays a major role in behavior stimulated by rewards. "This protein acts like a sensor for dopamine," Jasanoff said.
In their latest work, Jasanoff and his colleagues injected BM3h-9D7 into the brains of rats to measure dopamine activity in the ventral striatum, a reward-processing brain region. "We then gave the animals little electrical jolts to that part of the brain, stimuli much like what one might see in drug addiction," Jasanoff said. [Inside the Brain: A Photo Journey Through Time]
The scientists generated a 3D map of dopamine release in the brains of the rodents.
"We showed dopamine release in the nucleus accumbens core, a brain region we knew was a target for dopamine, but seeing it as the brightest spot in the brain map was a new finding, one consistent with some previous results," Jasanoff said. "Our sensor also found evidence of dopamine release in parts of the brain not usually studied in invasive studies."
Although scientists did previously have ways of analyzing molecular activity in the brain, these are typically invasive strategies involving either cadaver brains or small portions of living brains. "Here, we're getting the best of both worlds — the level of detail one can get with invasive strategies and the chance to look at the whole brain with noninvasive strategies such as fMRI," Jasanoff said.
Jasanoff did caution that since BM3h-9D7 latches onto dopamine, it might somewhat disturb normal dopamine function in the brain, potentially skewing results. Still, he said the levels of BM3h-9D7 they use are much lower than the concentrations of dopamine at the synapses, the gaps between neurons where brain cells do most of their communication with each other.
The research team is now working on sensor proteins that bind to other neurotransmitters such as serotonin. However, there is currently no way to use several of these sensor proteins at the same time to track multiple neurotransmitters simultaneously. Essentially, these sensor proteins all look the same to fMRI, so researchers have no way of distinguishing the effects of one from another.
"You can think of them as not being different colors, but all the same color, so you can't tell them apart if you use more than one at once," Jasanoff said.
The researchers now aim to improve the sensitivity of their technique. "This can't be used on humans yet," Jasanoff said.
Ultimately, "we hope to use these sensors to study and help develop better models of the brain, such as models of how the brain behaves when learning a task, or better models of addiction," Jasanoff said. "Once we have better models of addiction, perhaps we can test treatments for addictions to different drugs."
The scientists detailed their findings in tomorrow's (May 2) issue of the journal Science.
Follow us @livescience, Facebook & Google+. Original article on Live Science.
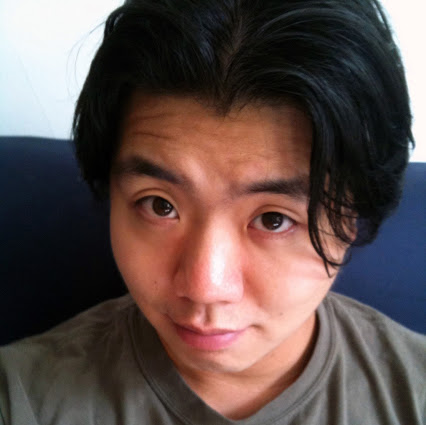