Weird 'Entangled' Light Gives Microscope Sharper Images
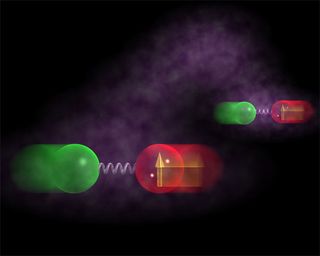
The first microscope that uses the eerie trick of quantum entanglement to increase its sensitivity has been developed by Japanese researchers.
The new tool relies on a weird principle of quantum mechanics, in which two particles can become entangled so that even when separated by large distances, say light-years, they are intimately connected. Using such entangled photons, or particles of light, the microscope reveals things that are completely transparent, visualizing them in a much better quality than could be done with ordinary light.
Physics guru Albert Einstein once famously called it "spooky action at a distance."
This unique property is already being looked at as a potential mechanism for quantum information technologies, such as quantum cryptography and quantum computation. But a group of scientists from Hokkaido University in Japan decided to go further, and demonstrated that quantum entanglementcan also be used in fields such as microscopy. [Magnificent Microphotography: 50 Tiny Wonders]
Most microscopes are limited in their resolving power by what is known as the Rayleigh diffraction limit —which states that it is impossible to image objects that are smaller or closer together than the wavelength of light used in the microscope to illuminate them.
Creative microscopy
The idea of using entangled photons to beat this limit was first suggested in a theoretical paper by physicist Jonathan Dowling and his colleagues at Louisiana State University in 2001.
Sign up for the Live Science daily newsletter now
Get the world’s most fascinating discoveries delivered straight to your inbox.
Now Shigeki Takeuchi and his team have actually created such a microscope. To accomplish this, as they detail in their paper in Nature Communications, they first generated entangled photons by converting a laser beam into pairs of photons that were in opposite polarization states (which describes how light waves are oriented) at once. (Quantum particles can be in two states at once — something called superposition.) The physicists used special nonlinear crystals to achieve the superposition of the photons' polarization states, which in this case were horizontal and vertical. The two photons in the pair would be considered entangled, and an action on one of them should affect the other regardless of the distance between them.
The researchers then focused the entangled photons on two adjacent spots on a flat glass platewith a Q-shaped pattern made in relief on the plate's surface. This pattern is only 17 nanometers higher than the rest of the plate — something that is very difficult to see with a standard optical microscope.
Entangled photons, however, significantly improve the visibility of this pattern. The Hokkaido University researchers say the signal-to-noise ratio, which describes roughly how sharp the image is, is 1.35 times better than the standard quantum limit when using their technique. And the resulting image is noticeably improved, simply by visual inspection. [Wacky Physics: The Coolest Little Particles in Nature]
"An image of a Q shape carved in relief on the glass surface is obtained with better visibility than with a classical light source," the researchers write in their paper.
In order to construct the images, the tiny differences in optical path length, which involves the time it takes light to pass through a material, between the two beams were detected using interference. The differencein optical path length was due to the tiny difference of the thickness of the glass. When both beams hit a flat part of the surface, they traveled the same distance and created a corresponding interference pattern. But when they hit areas of different heights, the interference pattern was different.
The scientists then analyzed the shape of the surface by analyzing the change in the interference pattern and measuring the difference in the phase of the light between the two photon states when the spots moved across it. Measuring this difference with entangled photons is much more precise, because a measurement on one entangled photon provides information about the other, so together they provide more information than independent photons, resulting in the larger detection signal and sharper image.
As a result, with the same number of photons, the signal-to-noise ratio using entangled photons is better than that with ordinary light.
Importance for biology
One classical way to image smaller objects without using entangled photons is to use shorter and shorter wavelengths of light. This way, one could improve resolution by switching from visible light to X-rays. But X-ray microscopesare difficult to use and coherent X-ray sources like X-ray lasers, in which the light waves have matching phases, are very difficult and expensive to build, said Dowling, who was not involved in the study. [Images: Small Worlds Come to Life in Stunning Photos]
"The quantum entanglement idea gives a road to get X-ray resolution using only visible light. In the future, this could lead to inexpensive microscopes that use ordinary lasers to get this resolution," Dowling said.
Physicist Jonathan Matthews of the University of Bristol in the U.K., who also was not involved in the research, said the main achievement is the demonstration that refractive index microscopes can be fundamentally enhanced.
The Japanese scientists said their research is especially important for applications in optics and biology. "It is a very powerful tool to investigate transparent samples such as biological tissues, and, in particular, living cells, without them being damaged by intense probe light," Takeuchi said.
Dowling agreed. "If you're imaging living organisms in situ, the X-rays may kill or damage the organism, but the wavelengths in [this] experiment are in the infrared and so would not harm the organism."
There are challenges, however. The biggest one is that the entangled photon light sources currently available are very faint, said Dowling, and while they give the improved resolution, the rate at which the image is acquired is very slow. "In this experiment the entangled photons arrive at about 5 photons per second. It's likely that to produce the image [shown above] they had to wait hours or days," he said.
"To be a marketable technology, a much brighter source of entangled photons must be developed, as biologists and doctors are unlikely to be prepared to wait hours for an image to form."
Follow us @livescience, Facebook & Google+. Original article on Live Science. Follow the author on Twitter @SciTech_Cat.