'Once again, innovation and proliferation ended with catastrophe': The environmental disaster of plants taking over the world
"By colonizing the continents and moving to the source of the elements whose availability constrained their ocean-dwelling ancestors, land plants set themselves up to become the second great world-changers."
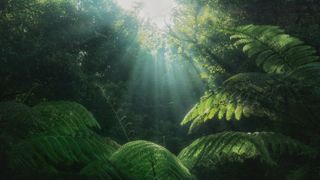
The excerpt below is taken from "Elemental: How Five Elements Changed Earth’s Past and Will Shape Our Future" (Princeton University Press, 2023) by Stephen Porder. It looks at how one of the biggest events in Earth's history came to be: plants colonizing the continents.
Plants are so ubiquitous on land that it's hard to imagine their absence, but for almost 90% of Earth's history, there was no life on land, or at the very least no plants. Land plants emerged a little more than 400 million years ago, which compared with the 4-billion-year history of life on Earth, is comparatively recent.
This evolutionary leap allowed them to become (arguably) only the second group of organisms to radically change the world, a full 2 billion years after the first, cyanobacteria, oxygenated the planet. Their world-changing predecessors precipitated a Great Oxidation Event that was likely the biggest environmental disaster in history — but also set the stage for all multicellular life on Earth. Land plants did not have quite this big an impact, but theirs was greater than any other group of organisms in the intervening two billion years.
For reasons I'll explore below, plants' evolutionary innovations are, in some ways, best understood through their connection to their cyanobacterial predecessors, and to the next group of world-changing organisms to evolve — humans. That connection lies in Life's Formula, the five elements that make up all living things: hydrogen, oxygen, carbon, nitrogen and phosphorus.
Let's set the stage for the story of plants by considering the world into which they emerged. The oceans of 400 million years ago were not radically different from those that cover 70% of Earth's surface today. Earth was much warmer, however, with a tropical climate from pole to pole. All the major types of life, including vertebrate and invertebrate animals of all shapes and sizes, roamed the seas. The continents peeking out from the watery surface were about their modern sizes, though not in their modern locations. Crucially, the chemistry of the ocean was similar to today, and understanding that chemistry helps explain how truly extraordinary, and world changing, the move to land was.
How did organisms live in that ancient ocean? As today, ocean food chains were built on the consumption of oxygen-producing photosynthetic organisms like cyanobacteria and plankton. The cellular machinery of photosynthesis in these single-celled organisms was sustained by nitrogen inputs from cyanobacteria and other microorganisms that could "fix" nitrogen from the limitless supply in the air.
Water (hydrogen plus oxygen) and nitrogen are three elements in Life's Formula, the elements that all living things share in very similar ratios. Sunlight, abundant water and "fixed" nitrogen fueled the acquisition of a forth — carbon — through photosynthesis. Despite the fact that cyanobacteria can tap into a virtually limitless source of nitrogen from the air, we think nitrogen placed a key limitation on how much life existed in the ancient ocean (that constraint remains today). It is a bit of a puzzle as to why this would be the case. Nitrogen fixation may give cyanobacteria a leg up on photosynthetic organisms that cannot pull off this remarkable bit of biological alchemy. But once a cyanobacteria cell dies and decomposes, the nitrogen it captured should become available to other organisms. Recycling is the norm in nature — once a scarce nutrient enters a system, it tends to stay there, fiercely sought after by all concerned. So why, when cyanobacteria could tap into a virtually limitless bank account of nitrogen in the air, did nitrogen remain relatively scarce in the ocean? Why didn't the cyanobacteria cause it to accumulate until it was no longer in short supply?
Sign up for the Live Science daily newsletter now
Get the world’s most fascinating discoveries delivered straight to your inbox.
This puzzle has preoccupied scientists in my field for decades, and like many good puzzles there is no single, clear answer. Nitrogen losses are clearly important, but I want to focus on another among the many reasons people have come up with: that the proliferation of cyanobacteria specifically, and photosynthetic organisms in the ocean in general, was limited by another element in Life's Formula.
The most abundant two elements in Life's Formula are hydrogen and oxygen. Living in the ocean, cyanobacteria had plenty of access to these. Photosynthesis uses sunlight and water efficiently to capture carbon, of which there is no shortage in the ocean. Research from as early as the 1950s showed convincingly that enough CO2 gas dissolves into the ocean that it rarely, if ever, is a constraint to growth. Photosynthetic machinery requires lots of nitrogen, but cyanobacteria can fix nitrogen, which dissolves in ocean water since it is so abundant in the air. And then … there's phosphorous.
It turns out that organisms that can fix nitrogen tend to have high demands for other atoms — particularly phosphorus, but also iron and molybdenum. The latter two are important components of the biological machine (the nitrogenase enzyme) that carries out nitrogen fixation. Phosphorus, iron, and molybdenum, unlike nitrogen, are virtually absent from the air. They are made available to organisms by the chemical breakdown of rock, and thus, with an admitted lack of linguistic imagination, scientists call them "rock-derived." We now think that these rock-derived elements limit the growth of cyanobacteria and other nitrogen-fixing organisms in the oceans. Thus, while life might have been proximately limited by the amount of nitrogen, the amount of nitrogen those organisms could capture was ultimately limited by the supply of elements derived from the weathering of rocks.
Imagine yourself as a single-celled, photosynthetic organism floating in the middle of the ocean 400 million years ago, more than 1,000 miles from land. If you're at the surface, there is plenty of sunlight available to drive photosynthesis. There are plenty of water molecules to split using the energy from the sun. If you're a nitrogen fixer, like cyanobacteria, you can build the machinery to capture nitrogen gas that is dissolved in the water. But where do you get the elements — the rock-derived phosphorus, iron, and others — needed to build that machinery? Not from the weathering of rocks at the ocean bottom — those are miles down — and even if you managed to get down there, there wouldn't be any light to fuel photosynthesis. As a single-celled organism in the upper ocean, you would just have to wait and hope that those elements come to you.
But if you're an unlucky single-celled organism, you live in a vast ocean desert. These places have very little life, despite being replete with sunlight and CO2, because they lack the other elements of Life's Formula. The only source of rock-derived phosphorus, for example, is the transport of material from the continents — a slow trickle of dirt from rivers and dust falling on the ocean surface. Floating in the middle of the Paleo-Pacific Ocean, you are at the mercy of the currents. There are no rocks for miles: up, down, or sideways. There is nothing you can do to increase your access to rock-derived elements. No way to access the fifth-most abundant element in your cells — phosphorus — and the other atoms derived from the breakdown of rocks. No way, that is, except to evolve and move to the source: land.
As with the cyanobacterial revolution that oxygenated the planet, the evolutionary innovations that allowed plants to complete the slow march landward revolved around access to the elements in Life's Formula. A first, and critically important, step was to bring the photosynthetic machinery from the ocean with them. The chloroplasts in plant leaves — the place where photosynthesis occurs — have their own DNA. It's the DNA of photosynthetic ocean bacteria that, long ago, merged into plant cells. Chloroplasts are thus an example of endosymbiosis — an organism within an organism. As a result of this endosymbiosis, the chemical reaction of plant photosynthesis is the same as cyanobacteria photosynthesis. It uses the same machinery. That is why land plants pump out oxygen during photosynthesis in the same way cyanobacteria do.
Living in the ocean meant using water for photosynthesis wasn't a problem. But on land, the need for water means a constant struggle to stay hydrated. The struggle is encapsulated by Life's Formula, which starts with hydrogen and oxygen. Because land plants inherited their photosynthetic machinery from their ocean-dwelling, single-celled ancestors, they use the same hyper-efficient, water-reliant photosynthesis. They split water using the energy from sunlight, capture CO2, and produce sugars to build their cells (and oxygen, by evolutionary accident). But every moment they open their leaves tiny pores to let CO2 diffuse in from the air they lose scarce water out through the same conduit. This is a scarcity ocean-dwellers don't have to deal with.
The evolutionary solution to this scarcity was the development of water saving mechanisms: leaf waxes, extensive root networks, and symbiosis with fungi that explored every nook and cranny of the soils. These innovations gave access to water, and as roots and fungi attacked the rocks below, they liberated phosphorous as well. These rocks were far out of reach for plants' ocean-dwelling predecessors, but right beneath their "feet" on land. By chemically and physically attacking the rocks upon which they grew, plants and their fungal partners became the world's first, and most efficient, miners, and gained greater access to the key elements in Life's Formula.
By colonizing the continents and moving to the source of the elements whose availability constrained their ocean-dwelling ancestors, land plants set themselves up to become the second great world-changers. To understand how, we have to move from understanding the paleo-ocean to understanding the paleo atmosphere. As today, nitrogen (as N2 gas, two nitrogen atoms bound so tightly together they are virtually inert) and oxygen (as O2 gas, two oxygen atoms bound together loosely enough to be very reactive) made up the vast majority of the air. But the best available evidence suggests CO2 levels may have been ten times higher than today, and the heat trapped by all that CO2 meant the world was very hot, probably about 10 degrees Fahrenheit (5.5 degrees Celsius) hotter than today. This may not sound like a lot, but such a world was hot enough to have no ice at either pole, the northern one covered by bathtub-temperature ocean and the southern by the supercontinent Gondwana.
Land plants made three key innovations. First, they found a new way to capture sunlight and thus carbon. In this case the innovation wasn't a new biochemical reaction but the movement of this reaction to a new place. Second, they evolved a way to withstand water scarcity on land by building root networks and partnering with fungi (among other things). Finally, they became miners, digging for critical rock derived nutrients that were, and remain, scarce in the ocean. Their innovations in getting water and nutrients allowed their wild proliferation. Proto-forests spread across much of the supercontinent that spanned from equator to pole. But, as with cyanobacteria, the story of plants also shows how unprecedented access to life's essential elements can have consequences. Once again, innovation and proliferation ended with catastrophe.
The catastrophe came about because the elements in Life's Formula are also contained in the greenhouse gases that regulate Earth's climate. As today, 400 million years ago the main gas keeping the planet warm was CO2. When plants evolved, they pulled CO2 from the air to build their tissues, and when those tissues died, some of that carbon got stuck in soils. Withdrawal #1 from the bank of CO2 in the air. Plants also accelerated the dissolution of minerals on land, which had the net effect of removing CO2 from the air and storing it on the ocean floor as limestone. Withdrawal #2. Finally, geologic conditions allowed the growth and repeated flooding of the vast lowland swamp forests that emerged during what is, not coincidentally, known as the Carboniferous Period. When plants growing in those swamps died, their remains were protected from decomposition. Their burial, over millions of years, represented yet another net transfer of CO2 out of the air. Withdrawal #3. All else being equal, you can't increase the rate at which you withdraw from a bank account without having that account go down. With the triple whammy of withdrawals that land plants imposed, the amount of CO2 in the air began to fall.
Eventually, plants' innovations pulled enough CO2 out of the air that the greenhouse effect began to weaken. The pan-tropical Earth, which had supported great forests across most of its land, began to cool. It is unclear how long the process took before Earth chilled enough to have ice ages. But by 300 million years ago, roughly 100 million years after plants got going in earnest on land, Earth had cooled enough that the vast tropical forests were gone from most of the planet. They were frozen by their own success. An environmental disaster spurred by new access to the elements in Life's Formula, subsequent proliferation, and collateral consequences.
The process driven by plants was slow: a drip, drip, drip out of the bank account of CO2 in the air and a transfer of that carbon below ground. Some of that carbon was gradually compressed, concentrated, and turned into coal. Then, 300 million years after those tropical trees succumbed to environmental changes of their own making, the next world-changing organism, humans, discovered that carbon-rich bank account.
We began burning this stored carbon at a rate never before seen in the history of our planet. We used the energy that burning produced to build dams and capture water, allowing us and our crops to stay hydrated on land. We used that energy to industrially fix nitrogen and mine phosphorus to fertilize our now-irrigated farms. And we too, are changing the world, even faster than our predecessors. But like them, our success, and environmental peril, is tied inextricably linked to the elements in Life's Formula.
Text from ELEMENTAL by Stephen Porder. Copyright © 2023 by Princeton University Press. Reprinted by permission of Princeton University Press.
Elemental: How Five Elements Changed Earth’s Past and Will Shape Our Future - $22.39 at Amazon
An ecologist explores how life itself shapes Earth using the elemental constituents we all share.
Stephen Porder is associate provost for sustainability and professor of ecology, evolution, and organismal biology at Brown University. He is also a fellow in the Institute at Brown for Environment and Society. His writing has appeared in the New York Times, Natural History, and other leading publications. He is cofounder of Possibly, which airs on The Public's Radio and provides practical advice on sustainability to a general audience.