Energy of '25 billion atomic bombs' trapped on Earth in just 50 years, all because of global warming
A new study has revealed that 380 zettajoules of energy was trapped by global warming between 1971 and 2020.
Global warming has trapped an explosive amount of energy in Earth's atmosphere in the past half century — the equivalent of about 25 billion atomic bombs, a new study finds.
In the paper, published April 17 in the journal Earth System Science Data, an international group of researchers estimated that, between 1971 and 2020, around 380 zettajoules — that is, 380,000,000,000,000,000,000,000 joules — of energy has been trapped by global warming.
Such a big number is hard to put into context. But two researchers, who were not involved in the study, have put it into perspective by comparing the energy to that released by nukes. However, even then, the amount is still hard to wrap your head around.
In an article for The Conversation, Andrew King, a climate scientist at the University of Melbourne in Australia, and Steven Sherwood, a climate scientist at the University of New South Wales in Sydney, calculated that 380 zettajoules is equivalent to around 25 billion times the energy released during the detonation of "Little Boy," the atomic bomb dropped on Hiroshima, Japan, on Aug. 6, 1945.
Even more mind-blowing, the energy absorbed by the planet during this time period likely equates to only around 60% of total greenhouse gas emissions, so the actual number is even higher, King and Sherwood wrote.
Related: Climate 'points of no return' may be much closer than we thought
But such a large amount of energy is also puzzling, because based on that amount of heat being trapped in the atmosphere, the average global temperature should have risen by dozens of degrees since preindustrial times, rather than by the 2.2 degrees Fahrenheit (1.2 degrees Celsius) that we have observed, the pair wrote. So where has all this extra energy gone?
Sign up for the Live Science daily newsletter now
Get the world’s most fascinating discoveries delivered straight to your inbox.
According to the study, the oceans have absorbed around 89% of the energy (338.2 zettajoules), land has absorbed 6% (22.8 zettajoules), 4% (15.2 zettajoules) has melted parts of the cryosphere — the part of Earth's climate system that includes snow, sea ice, freshwater ice, icebergs, glaciers and ice caps, ice sheets, ice shelves and permafrost — and just 1% (3.8 zettajoules) has remained in the atmosphere.
The majority of the heat absorbed by the seas is trapped in the upper 0.6 mile (1 kilometer) of the oceans. This has spared humanity from the brunt of climate change so far, but it has also caused massive increases in sea surface temperatures, which has accelerated polar melting, damaged marine ecosystems, increased the severity of tropical storms and begun to disrupt ocean currents.
However, the oceans will not protect our planet forever, King and Sherwood wrote, so we must begin rapidly decreasing greenhouse gas emissions by decarbonizing the global economy to ensure our future survival. "We're in a race, and the stakes are as high as they could possibly be — ensuring a liveable climate for our children and for nature," they wrote.
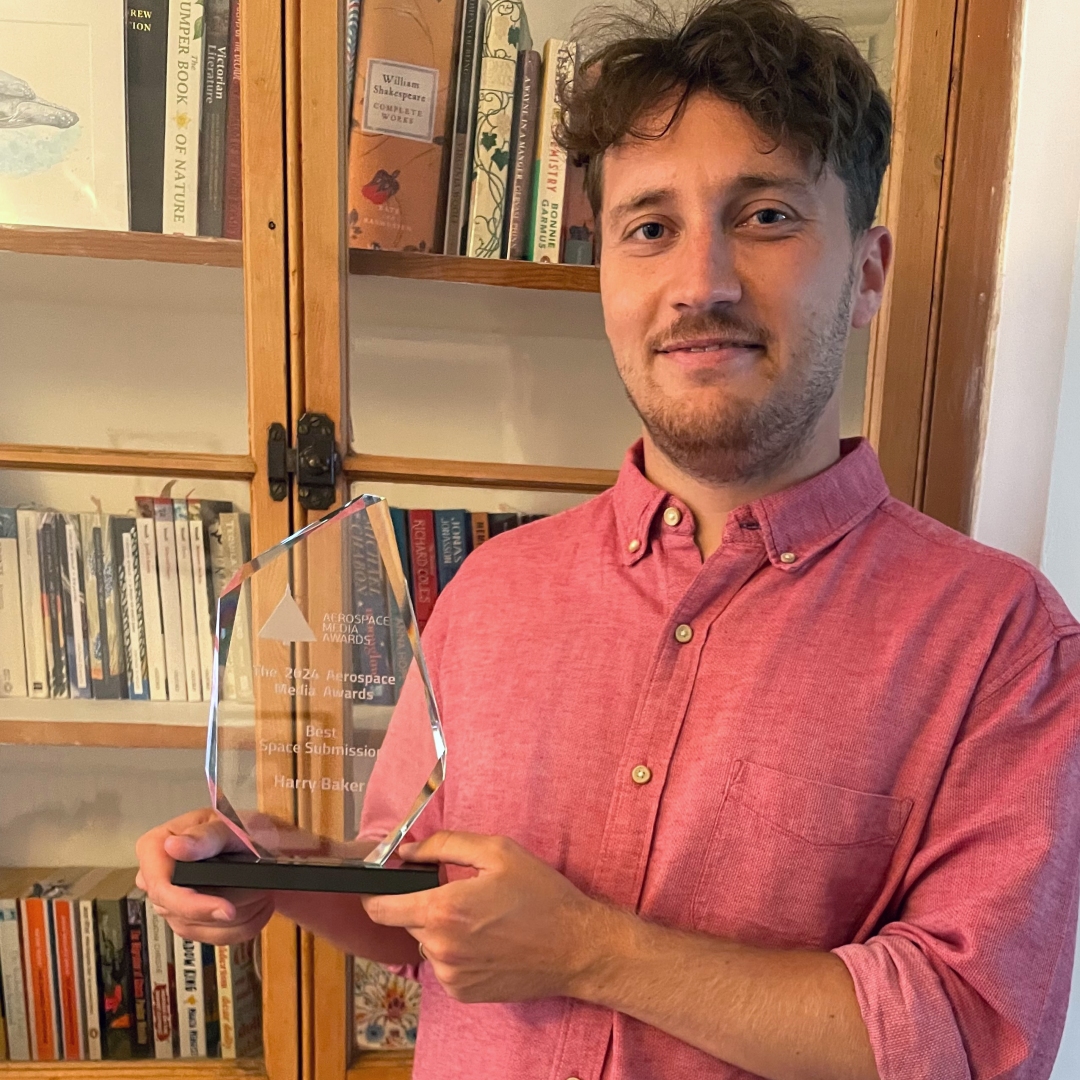
Harry is a U.K.-based senior staff writer at Live Science. He studied marine biology at the University of Exeter before training to become a journalist. He covers a wide range of topics including space exploration, planetary science, space weather, climate change, animal behavior and paleontology. His recent work on the solar maximum won "best space submission" at the 2024 Aerospace Media Awards and was shortlisted in the "top scoop" category at the NCTJ Awards for Excellence in 2023. He also writes Live Science's weekly Earth from space series.