'Ghost particles' detected inside the Large Hadron Collider for the first time
Ghostly neutrinos have never been seen inside a particle accelerator, until now.
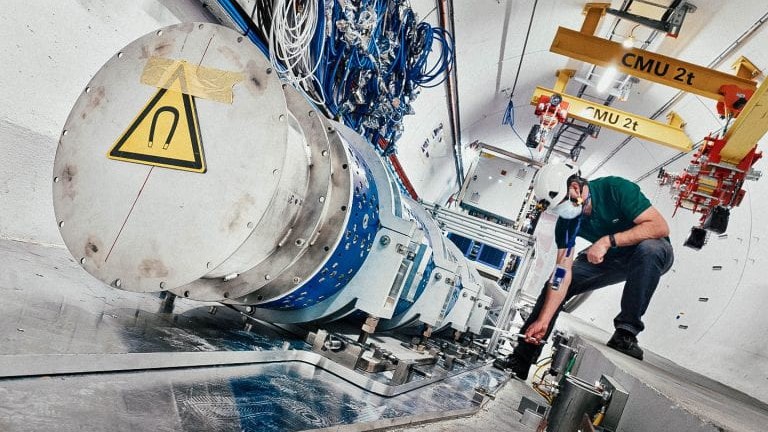
Physicists have detected "ghost particles" called neutrinos inside an atom smasher for the first time.
The tiny particles, known as neutrinos, were spotted during the test run of a new detector at the Large Hadron Collider (LHC) — the world's largest particle accelerator, located at CERN near Geneva, Switzerland.
The landmark discovery, made by CERN's Forward Search Experiment (FASER) collaboration and presented in a Nov. 24 paper in the journal Physical Review D, is not just the first time that neutrinos have been seen inside the LHC, but it's also the first time they've been found inside any particle accelerator. The breakthrough opens up a completely new window through which scientists can investigate the subatomic world.
Related: Beyond Higgs: 5 Elusive Particles That May Lurk in the Universe
"Prior to this project, no sign of neutrinos has ever been seen at a particle collider," study co-author Jonathan Feng, a physics professor at the University of California, Irvine and co-leader of the FASER collaboration, said in a statement. "This significant breakthrough is a step toward developing a deeper understanding of these elusive particles and the role they play in the universe."
Every second, about 100 billion neutrinos pass through each square centimeter of your body. The tiny particles are everywhere — they're produced in the nuclear fire of stars, in enormous supernova explosions, by cosmic rays and radioactive decay, and in particle accelerators and nuclear reactors on Earth.
But despite their ubiquity, the particles remain hard to catch. Because neutrinos have no electrical charge and almost zero mass, they barely interact with other types of matter. True to their ghostly nickname, neutrinos view the universe's regular matter as incorporeal, and they fly through it at close to the speed of light.
Sign up for the Live Science daily newsletter now
Get the world’s most fascinating discoveries delivered straight to your inbox.
Just because they're hard to catch doesn't mean that neutrinos can't be caught, however. Some of the most famous neutrino detection experiments — such as Japan's Super-Kamiokande detector, Fermilab's MiniBooNE, and the Antarctic IceCube detector — have all detected solar-generated neutrinos indirectly through an effect called Cherenkov radiation. Just as a plane traveling faster than the speed of sound creates a sonic boom, a particle traveling through a light-slowing medium (like water) faster than light is able to creates a faint blue glow in its wake. By looking for this glow, scientists are able to spot the trails of particle byproducts created after neutrinos strike an atomic nucleus dead-on.
But while experiments like these are great for detecting the signatures of neutrinos that stream through Earth from the sun, they still leave scientists with very little insight into the types of high-energy neutrinos produced when particles smash into each other inside particle accelerators. To find these homegrown neutrinos, the scientists at the FASER collaboration created a new detector called the FASERnu.
The FASERnu is like a particle-detecting s'more, made up of dense metal plates of lead and tungsten that sandwich multiple layers of light-detecting gunk called emulsion. First, the neutrinos crash into the atomic nuclei in the dense metal plates to produce their particle byproducts. Then, according to Feng, the emulsion layers work in a similar way to old-fashioned photographic film, reacting with the neutrino byproducts to imprint the traced outlines of the particles as they zip through them.
By "developing" the emulsion and analyzing the particle trails left behind, the physicists figured out that some of the marks were produced by neutrinos; they could even determine which of the three particle "flavors" of neutrino — tau, muon or electron — they had detected. This confirmed that they had not only picked the right spot inside the gigantic 17-mile (27 kilometers) ring to detect neutrinos, but that their new detector was actually able to see them.
Now that they've struck upon a winning detector, the physicists have started building an even bigger version of it, which they say will not only be a lot more sensitive to spotting the elusive particles, but will also be able to detect the difference between neutrinos and their antimatter opposites, antineutrinos. When the LHC powers up again in 2022, they plan to use the detector to study the neutrinos produced by the particle accelerator in-depth.
"Given the power of our new detector and its prime location at CERN, we expect to be able to record more than 10,000 neutrino interactions in the next run of the LHC, beginning in 2022," Casper said. "We will detect the highest-energy neutrinos that have ever been produced from a human-made source."
Neutrinos aren't the FASER scientists' only quarry, either. The team is also working on an experiment to detect hypothetical "dark photons," which physicists think could be intimately connected to dark matter, the mysterious, non-luminous substance believed to account for roughly 85% of the matter in the universe.
Originally published on Live Science.
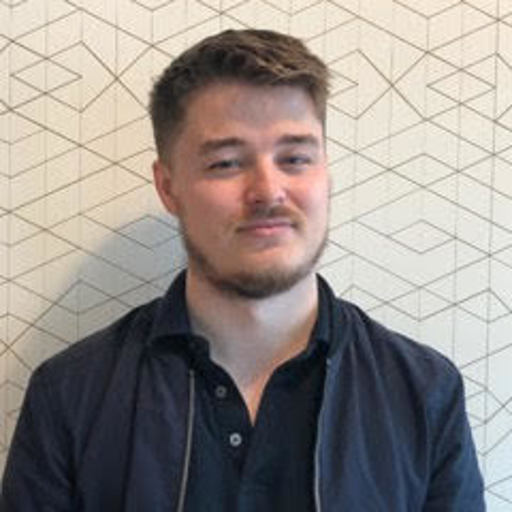
Ben Turner is a U.K. based staff writer at Live Science. He covers physics and astronomy, among other topics like tech and climate change. He graduated from University College London with a degree in particle physics before training as a journalist. When he's not writing, Ben enjoys reading literature, playing the guitar and embarrassing himself with chess.