
Where Is the Rest of the Universe?
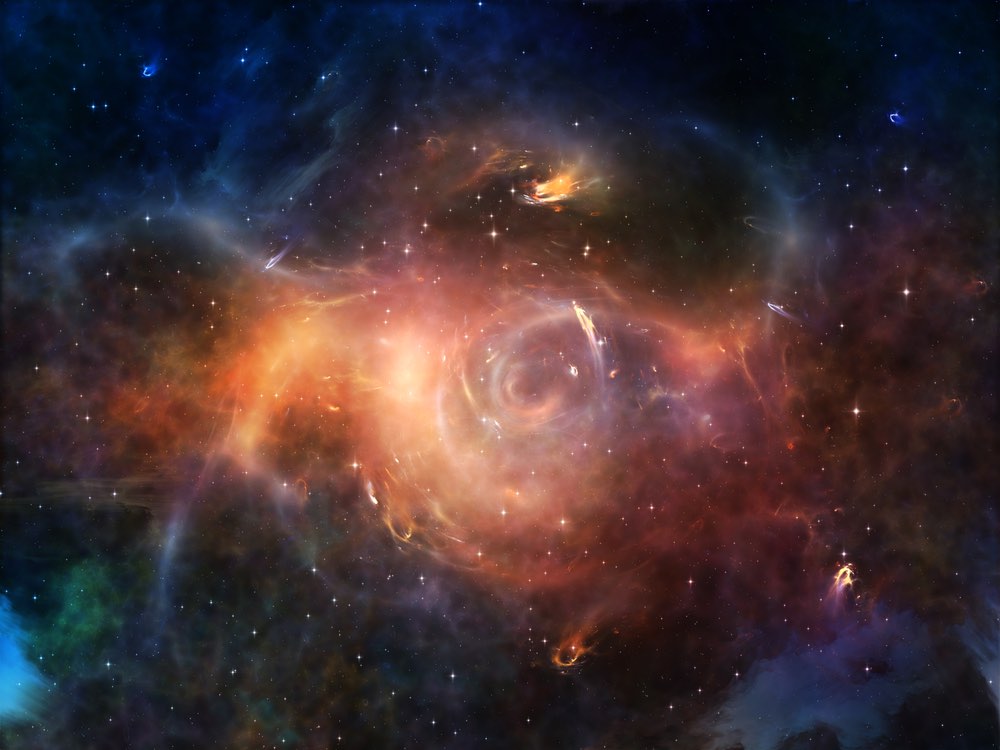
Most of the universe is made up of "stuff" that is invisible, possibly intangible and interacts with other things only via the force of gravity. Oh, yes, and physicists don't know what the stuff is or why it makes up so much of the universe — some four-fifths of its mass.
They call it dark matter.
So where is this mysterious stuff that makes up such a huge chunk of our universe, and when will scientists find it?
First, though, how do we know it's even out there?
Dark matter was first hypothesized in the 1930s when Swiss astronomer Fritz Zwicky realized that his measurements of the masses of galaxy clusters showed some of the mass in the universe was "missing." Whatever was making galaxies heavier, it didn't give off any light, nor did it interact with anything else except via gravity.
Vera Rubin, in the 1970s, found that the rotation of galaxies was not following the predictions of Newton's laws of motion; the stars in galaxies (notably Andromeda) all seemed to be orbiting the center at the same speed, rather than those farther out moving more slowly as theories of gravity said they should. Clearly, something was adding mass to the outer parts of galaxies, something nobody could see. [Does the Universe Have an Edge?]
Other pieces of evidence came from gravitational lensing, which happens when the gravity of a large object bends the light waves around that object. Per Albert Einstein's theory of general relativity, gravity bends space (like a sumo wrestler might deform the mat he is standing on), so light rays bend around massive objects even though light itself is massless. Observations indicated that there wasn't enough visible mass to bend the light by as much as it was bending around certain galaxy clusters — in other words, the galaxies were more massive than they should be.
Get the world’s most fascinating discoveries delivered straight to your inbox.
Then there is the cosmic microwave background (CMB), the "echo" of the Big Bang, and supernovas. "What the CMB tells you is that the universe is spatially flat," said Jason Kumar, a professor of physics at the University of Hawaii. "Spatially flat" means that if you were to draw two lines across the universe, they would never meet, even if those lines were a billion light-years across. In a steeply curved universe, those lines would meet at some point in space.
Researchers then calculated how much matter the universe must have in order to be flat and produce the amount of normal matter (also called baryons) observed in the universe.
"I ask myself, 'Is the amount of matter I have equal to baryonic matter, and it's not," Kumar said.
There's now little dispute among cosmologists and astronomers that dark matter exists. Yet it seems unaffected by light, and it isn't charged like electrons or protons are. So far it has eluded direct detection.
"That's kind of the mystery," Kumar said. There are may ways scientists have tried to "see" dark matter – either via its interactions with normal matter or looking for particles that dark matter might become. "Those experiments are going to keep getting better, and don't seem to have any hiccups so far as they go to better detectors."
What we know it isn't
A number of theories have come and gone as to what dark matter is. One of the first was logical enough: The matter was hidden within massive astrophysical compact halo objects, or MACHOs, such as neutron stars, black holes, brown dwarfs and rogue planets. They don't emit light (or they emit only very little), so they are effectively invisible to telescopes. [The 9 Biggest Unsolved Mysteries in Physics]
Yet surveys of galaxies looking for small distortions in the light of background stars produced by a MACHO passing by — called microlensing events — couldn't account for the amount of dark matter around galaxies, or even a significant portion of it. "MACHOs seem as ruled out as ever," said Dan Hooper, an associate scientist at the Fermi National Accelerator Laboratory in Illinois.
Dark matter doesn't appear to be clouds of gas that telescopes wouldn't see, either. Diffuse gas would absorb light from the galaxies that are farther away, and on top of that, ordinary gas would re-emit radiation at longer wavelengths – there'd be a massive radiation of infrared light in the sky. Since that doesn't occur we can rule that out as well, Kumar said.
What it might be
Weakly interacting massive particles, or WIMPs, are some of the strongest contenders to explain dark matter. WIMPs are heavy particles — about 10 to 100 times heavier than a proton — that were produced during the Big Bang, though just small quantities are left today. These particles interact with normal matter via gravity or the weak nuclear force. More massive WIMPs would move more slowly through space, and therefore be "cold" dark-matter candidates, while lighter ones would move faster, and be "warm" dark matter. [Wacky Physics: The Coolest Little Particles in Nature]
One way to find them is in "direct detection" experiments, such as the Large Underground Xenon (LUX) experiment, which is a container of liquid xenon in a South Dakota mine. If a xenon nucleus seems to "bounce" with no explanation, that would be a candidate for getting hit with a dark-matter particle. The magnitude of the bounce would give an idea of the new particle's mass. But Hooper said that LUX hasn't seen anything yet.
Another way to see the WIMPs might be particle accelerators. Inside accelerators, atomic nuclei smash into each at near the speed of light, and in the process that energy of the collision gets turned into other particles, some new to science. So far, though, particle accelerators haven't detected anything that looks like a dark matter candidate, either.
Results from both direct detection and particle accelerators, however, have placed limits on the size and mass of this hypothetical dark-matter particle, Kumar said. The sensitivity of LUX is down to 200 MeV, or about a fifth of a proton's mass, and it could theoretically see particles as heavy as 1 TeV, which is comparable to some types of quarks. Since LUX saw nothing so far, that could well mean that dark matter isn't in that range.
Kumar said that it's possible that WIMPs are really heavy, and because they are so massive there just aren't that many of them, meaning the chance they'll hit a xenon atom is small.
Another possibility: axions. These subatomic particles could be detected indirectly by the kinds of radiation they emit as they annihilate or as they decay into other kinds of particles, or appear in particle accelerators. Yet there hasn't been any direct evidence of axions, either.
Since detecting heavy, slow-moving "cold" particles, like WIMPs or axions, hasn't yielded results yet, some scientists are looking at the possibility of lighter, faster-moving particles, which they call "warm" dark matter. There has been a renewed interest in such a dark-matter model after scientists found evidence of an unknown particle, using the Chandra X-ray Observatory, in the Perseus cluster, a group of galaxies about 250 million light-years from Earth. The known ions in that cluster produce certain X-ray emission lines, and in 2014, scientists saw a new "line" that may correspond to an unknown lightweight particle, Nicola Menci, an astrophysicist at Italy's National Institute for Astrophysics (INAF), told Live Science in an email.
If dark-matter particles are light, scientists are going to have a difficult time detecting them directly, said Tracy Slatyer, a physicist at MIT. Slatyer has proposed new kinds of particles that might make up dark matter.
"Dark matter with [a] mass below about 1 GeV is really hard to detect with conventional direct detection experiments, because they work by looking for the unexplained recoils of atomic nuclei… but when the dark matter is much lighter than the atomic nucleus, the recoil energy is very small," Slatyer said. Protons — a hydrogen nucleus — can't be any lighter than about 938 MeV, so a particle that weighs in the keV range would be 1,000 times lighter. "Think bouncing a ping-pong ball off a bowling ball; the bowling ball doesn't move very much," she said.
Slatyer said there is a lot of research being done on how to find dark-matter particles if current methods fail. Using "superfluid" liquid helium, semiconductors and even breaking of chemical bonds in crystals, are among the ideas being floated.
Kumar said one reason dark matter is so mysterious is precisely that physicists feel that they understand, to a point, how the Big Bang nucleosynthesis ― the origins of matter ― worked. The Standard Model, which predicted the Higgs boson, has been very successful so far, so unless they've all been really wrong about something fundamental it's odd that nobody has managed to detect a dark-matter particle yet.
If, for example, dark-matter particles are very different from what many current models predict, it's possible that particle accelerators wouldn't have seen it. Accelerators like the Large Hadron Collider are better at seeing things that interact with the strong nuclear force, which decay into other particles.
"If that's the way your dark matter works it's a great machine to find it," he said. "But if there isn't a heavier particle like that it's harder."
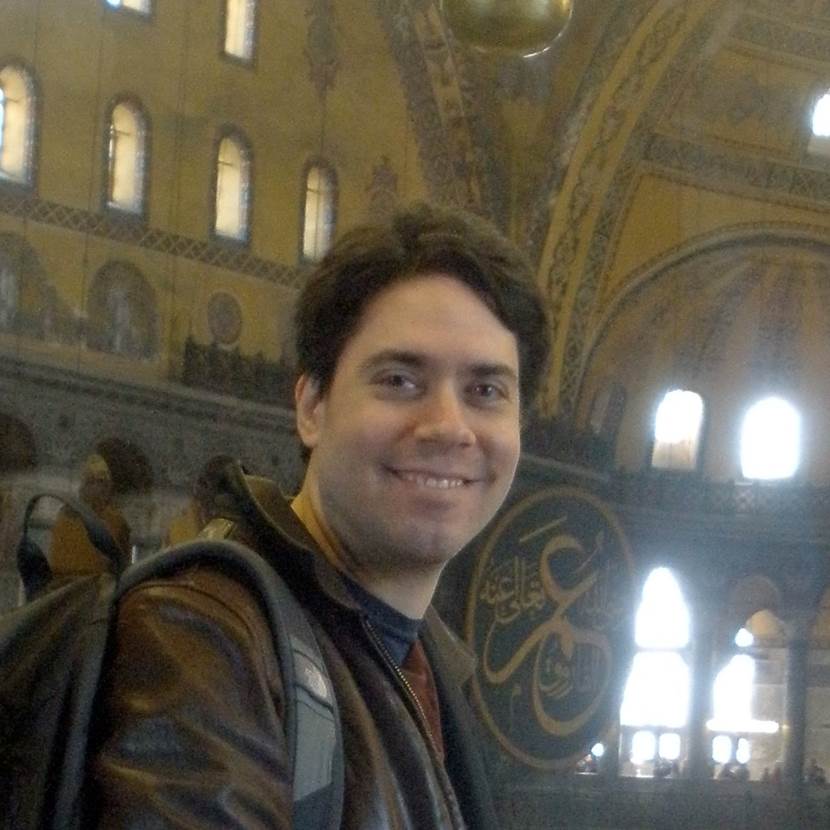