Dark matter hunter who found unexpected, giant 'Fermi bubbles' wins $100,000 physics prize
Tracy Slatyer, known for hunting dark matter in our galaxy and discovering evidence of an ancient Milky Way explosion, has won a $100,000 prize funded by tech billionaires.
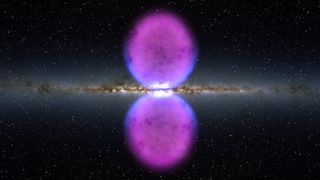
Editor's Note: This story was corrected at 12:30 p.m. E.D.T. to note that Slatyer received $100,000, not $50,000, for her work.
Tracy Slatyer, known for hunting dark matter in our galaxy and discovering evidence of an ancient Milky Way explosion, has won a $100,000 New Horizons Prize in Physics.
Slatyer, an MIT physicist originally from Australia, is most famous as a co-discoverer of the "Fermi Bubbles." While looking for hints of dark matter's signature in the gamma rays emanating from the center of the Milky Way, she and her colleagues found never-before-seen structures extending far above and below the galactic disk — aftershocks of a black hole outburst from millions of years ago that came to be known as "Fermi bubbles" after the Fermi Gamma-ray Space Telescope. But Slatyer is still hunting dark matter and has found promising (though still tentative) hints of the stuff at the galactic center.
The New Horizons award, given by the Breakthrough Prize Foundation each year, goes to "early career" researchers like Slatyer, who got her Ph.D. in 2010 and was hired at MIT in 2013. New Horizons prizes are smaller than the $3 million prizes Breakthrough hands out each year, typically to older and more established scientists. Slatyer was the only solo winner of a 2021 New Horizons prize in Physics, with the other two awards going to research teams of four members each. The prize money is donated by a group of tech billionaires (Sergey Brin, Anne Wojcicki, Mark Zuckerberg, Priscilla Chan, Yuri Milner, Julia Milner, Jack Ma and Pony Ma).
Breakthrough awarded Slatyer the prize "For major contributions to particle astrophysics, from models of dark matter to the discovery of the “Fermi Bubbles.”
Slatyer spends a lot of her time refining models of dark matter — working out precisely how its particles might behave and the implications of those different possibilities. And the rest of her time is spent hunting them down.
Related: The 12 strangest objects in the universe
Sign up for the Live Science daily newsletter now
Get the world’s most fascinating discoveries delivered straight to your inbox.
"It was a complete surprise," Slatyer told Live Science. "The prize wasn't even on my radar."
Gamma-ray traces of dark matter
About 84% of the mass in the universe is dark matter, which emits no light but exerts gravitational pull. Scientists don't know what that dark matter is or where it came from. But physicists can see indirect evidence for dark matter through its gravitational effects on luminous matter (the stuff we can see). The movements and arrangements of galaxies, the behavior of light across vast regions of space and the structure of the universe all suggest the presence of something out there that we can't directly detect — much of it clustered in "halos" around big galaxies like the Milky Way.
Right now, directly detecting dark matter is one of the biggest goals in physics. That's a large part of what Slatyer has spent her career trying to do. But so far, dark matter has been very good at hiding..
"If it interacts with the other forces we know about" — like electromagnetism, or the weak force and strong force in atomic nuclei — "it does so pretty weakly," Slatyer said.
But there's reason to think it does interact with other matter a little bit. The clue is in that number, 84%. Physicists tend to think about numbers in terms of "orders of magnitude." Meaning: Are they very small, small, medium, big, very big, very very big, or what? The precise number matters, but so does the general category it falls into. And 84%, from a physicist's perspective, is a medium-sized number. It's not clear why dark matter's portion of the universe should be medium-sized.
"Why is it kind of in the same ballpark as 50%?" Slatyer said. "Why isn't it 99.999%? Or 0.0001% percent of the matter in the universe?"
One possible explanation: Dark matter does periodically interact with other matter particles, but only faintly. There are other particles like this: Neutrinos are all around us, but they rarely interact and are only possible to detect with very sensitive equipment. In the early days of the universe, when everything was hotter and denser and closer together, those interactions happened all the time. The tight squeeze would have dramatically raised the odds of a dark matter particle running into another particle. But over time, the universe spread out and those interactions became less common.
There's a particular hypothetical interaction that does a good job of producing an 84%-dark matter universe in astrophysical models: If dark matter particles that collide with each other were to form particles of ordinary matter, that would lower the amount of dark matter in the universe over time. However, an interaction that can happen in one direction can also happen in the other, so luminous particles would sometimes collide to form dark matter.
Over time, Slatyer said, these two interactions would drive dark matter to an equilibrium, Slatyer said. Once you accept that general picture, you can calculate the properties of dark matter that would have led to our 84%-dark matter universe.
"If you do this calculation you find that it's pretty consistent with [what would happen] if dark matter were of a similar mass to the heaviest [luminous] particles ... and interacting with a strength comparable to the weak nuclear force," she said.
(In this framework, it probably interacts with the weak nuclear force. But physicists leave open the possibility that it interacts with an unknown force that's very similar to the weak force.)
When particles physicists do know about that mass range crash together, they produce photons (light particles) with tens or hundreds of gigaelectronvolts (GeV) of energy.
"And photons with tens or hundreds of GeV of energy we call gamma rays," she said.
If WIMPs are out there, occasionally bumping into each other, they should produce a faint gamma-ray glow that researchers might theoretically detect. Searching for those traces
That's why much of Slatyer's dark matter hunt relies heavily on data from NASA's Fermi Gamma-ray Space Telescope. One area where she hunts for gamma rays is in the nearest cluster of dark matter we know about: the densest part of the Milky Way's halo, right near its center.
The problem is, the center of the Milky Way has lots of other gamma-ray sources, some of which we don't know about. To identify the gamma rays coming from dark matter, researchers need to first model exactly what the gamma ray signal from the dark matter should look like. Then they need a "background model" — a detailed picture of all the other known gamma-ray sources coming from that part of the sky. That's all the stuff they have to ignore.
If Slatyer and her colleagues ever take a photo of the Milky Way's dark matter, they'll do it by studying huge numbers of gamma-ray photons detected from that part of space and subtracting out all the photons that can be explained without dark matter. Models suggest that solution should leave just a small remnant — measured in a few dozen individual gamma-ray particles over a decade of Fermi observations of the Milky Way — coming from dark matter interactions.
In the early 2010s, Slatyer and her collaborators thought they'd found that tiny excess. Careful study of Fermi data from the Milky Way center suggested a vague, bulbous shape in the gamma-ray spectrum.
"It looked almost like a fuzzy egg," she said.
That closely matched the expected picture of the Milky Way's halo.
But over time, they refined their image of that excess, like a camera slowly coming into focus. Eventually, they saw that the shape had sharp edges and a pinched waist, more like an hourglass than the expected fuzzy blob. The explanation: an outflow of luminous matter from the region around the black hole at the center of our galaxy, the trace of a blast in the last several tens of millions of years. They named these never-before-seen structures the "Fermi bubbles."
Related: 9 facts about black holes that will blow your mind
Now, Slatyer said, she and her colleagues are focused on a newfound excess of gamma rays discovered in Fermi data. It's faint, but does seem to closely match that fuzzy ball picture.
Again, however, researchers aren't sure whether they've stumbled onto a signal of dark matter or another never-before-seen background source. The excess does match the signal expected to come from colliding WIMPs, but it could also be explained by an unknown population of millisecond pulsars — tiny neutron stars whirling at incredible speeds. There are plenty of millisecond pulsars in the Milky Way, but this would require many more of them than expected, distributed around the galactic center.
Now, Slatyer said, the hunt is on for these pulsars. "Hot spots" in the gamma ray or radio-wave pictures of the sky, or any evidence of pulsing in the slim data, would suggest that once again the researchers accidentally discovered a new galactic object that isn't dark matter.
"If you showed me a dense population of pulsars in this region, then I'd be very happy in saying that the excess is from pulsars," she said.
If that discovery is coming, it might come in the next few years, thanks to MeerKAT, a radio telescope array in South Africa that should be able to find individual pulsars in that part of space. But proving instead that the excess is dark matter would be harder and take much longer.
"Obviously as a particle physicist I would be happy if it was dark matter," she said.
But it's possible, she said that this excess and the next one and the one after that turn out to be other features of the universe that no one had been looking for. That may not be dark matter, but it's still pretty great.
"That's one of the things I really like about this field," she said. "It's a little ungrateful to be like 'No, universe! You didn't give me the discovery I wanted!'"
Originally published on Live Science.