Physicists link 'quantum memories' in early step toward quantum internet
Two novel demonstrations bring the backbone of the quantum internet, quantum repeaters, a little closer.
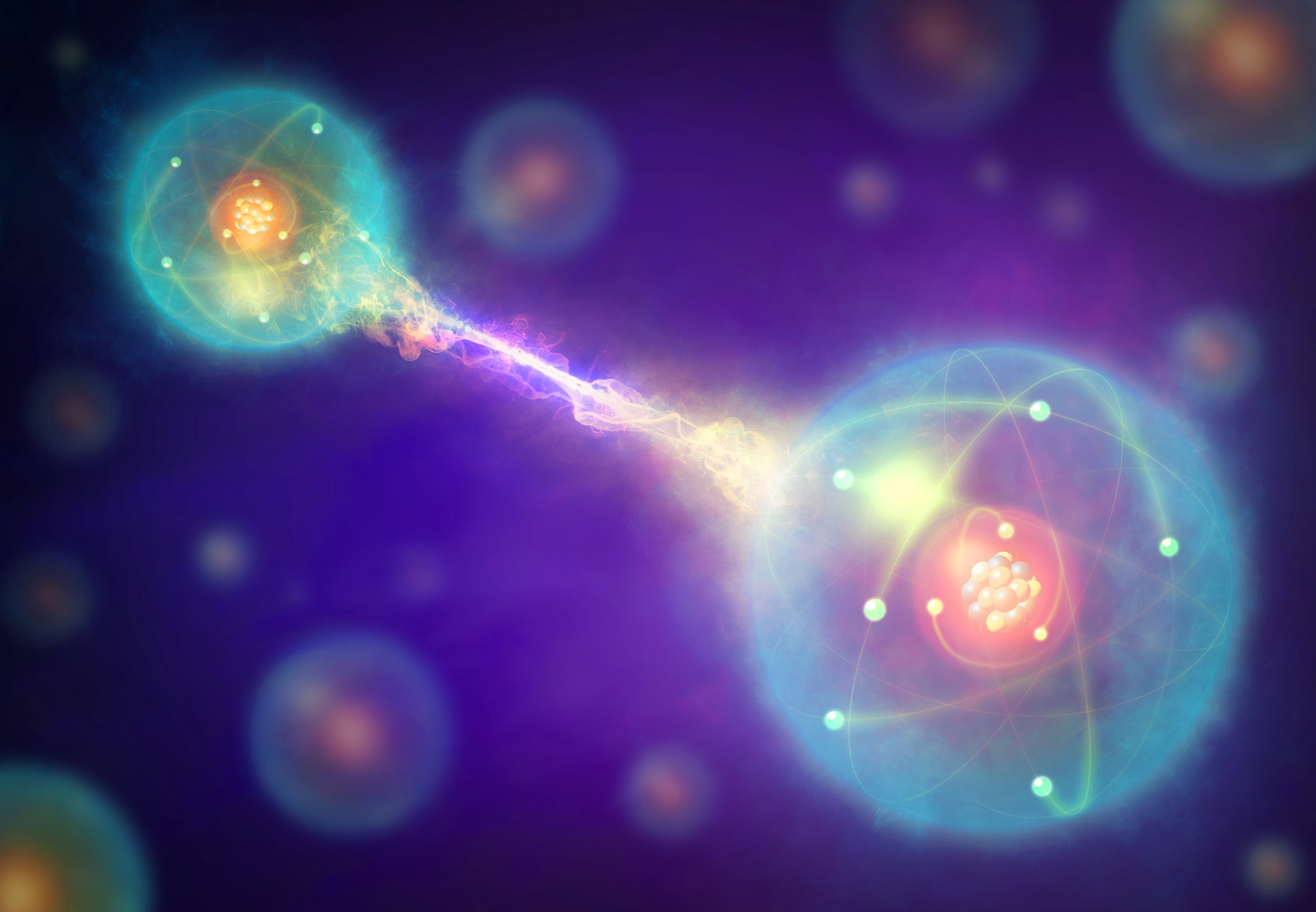
When the precursor to today's internet carried its first message in 1969, clunky but functional classical computers had already been around for decades. Now, physicists are designing the embryonic threads of a whole new internet for moving and manipulating a radically different type of information: the quantum bit, or "qubit." And this time, they aren't waiting for the corresponding computers to exist first.
Two teams have now demonstrated an ensemble of technologies essential to building the backbone of such a network — devices known as quantum repeaters. The researchers managed, for the first time, to use light particles to bind two crystals separated by tens of meters into a single quantum mechanical system and verify the connection in a simple way. The experiments foreshadow a future where institutions across the planet can take advantage of a bizarre type of connection called entanglement.
"This is for sure a new step for quantum repeater applications," said Julien Laurat, a physicist at Sorbonne University in France, who was not involved in the research.
Related: Infographic: How quantum entanglement works
Storing light in matter
One pillar of quantum information technology is the qubit, which is a system (like a particle) that exists in a combination of two states known as "superposition." The qubit's rich behavior compared with that of a classical bit (which can exist only as a 0 or a 1) allows for new modes of computation, somewhat like how a six-sided die is suited to different games than a two-sided coin.
In the recent experiments, teams at the University of Science and Technology of China (USTC) and the Institute of Photonic Sciences (ICFO) in Spain used photons, or light particles, to create qubits. Past experiments have often stored information of photons in gas clouds controlled precisely with lasers, but the USTC and ICFO researchers have advanced a new type of "solid state" quantum hard drive: glass crystals filled, or "doped," with ions of a rare-earth metal. The ions took the place of the gas in earlier experiments, and the glass held them in place.
"You can think of our doped crystals as pretty much being a frozen cloud," said Samuele Grandi, an ICFO physicist who worked on one of the experiments.
Sign up for the Live Science daily newsletter now
Get the world’s most fascinating discoveries delivered straight to your inbox.
When a photon enters the crystal, it crashes into the ions (which the researchers have carefully prepared to respond to the incoming particle) and transfers its energy to them. In that moment, the crystal is holding the photon's qubit and serving as a quantum memory, a storage device for quantum information.
A spooky connection
The second pillar of quantum communication is an ethereal link called entanglement, in which two particles or groups of particles act as one system, even while separated by large distances. This phenomenon lies at the heart of a quantum internet, yoking quantum devices much as fiber-optic cables and radio waves connect classical computers. A quantum network could stretch as far as one can entangle quantum memories, and no farther.
The problem is that, unlike bits on a hard drive, the ironclad rules of quantum mechanics forbid the copying and relaying of qubits in a quantum memory (a property that helps make quantum messages theoretically hack-proof). To overcome this obstacle, researchers imagine daisy-chaining quantum memories together with repeaters. To someday entangle memories between Boston and Washington, D.C., for instance, one might entangle the Boston memory with a memory in a New York repeater, and the New York repeater with the Washington, D.C., memory.
Grandi and his collaborators have taken a notable step toward such a device. Their apparatus starts with two laser-like devices, one on each side, , either of which can produce a pair of entangled photons. Even this first step is a challenge, with each device having just a 1-in-1,000 chance of doing so.
But with persistence, eventually one device will fire off twin photons. One photon goes straight into a corresponding quantum memory (the doped glass), and the other races down a fiber-optic cable. Halfway between the two devices (and their memories), this photon runs into a beam splitter — a material that lets the photon through half of the time.
That's where the quantum magic happens. When Grandi and his collaborators see a photon pop out of the beam splitter, they have no idea if it came from the right side or the left side. Therefore they have no idea whether the partner photon is living in the memory on the right or the memory on the left. Quantum mechanics give this uncertainty a profound consequence. Since the stored photon could be residing in the right memory or in the left memory, it must exist in a superposition of right and left, both present and absent in both memories in a way that entangles the two crystals together.
"The fact that you cannot know which way it came [from]," Grandi said, "this is what generates the entanglement between the memories that are now holding one photon between them."
When successful, the group's apparatus stored one photon between two entangled memories in neighboring labs, 10 meters (33 feet) apart — an outcome frequently described mathematically in quantum textbooks but rarely experienced in the real world.
"This, for me, was mind-boggling," Grandi told Live Science. "You know it works, but then you see it and this is really counterintuitive."
Crucially, the team could easily confirm the surreal connection. A photon emerging from the beam splitter means the memories are entangled. Researchers call this particle a heralding photon because it "heralds" entanglement. Other physicists have entangled quantum memories of various types before, but the ICFO and USTC experiments were the first to entangle crystal memories with this clear signal of entanglement.
The ICFO apparatus also used light of the same wavelength used in fiber-optic cables and proved that their memories could make multiple entanglement attempts at the same time — a step toward a quantum network carrying different messages simultaneously. The USTC group, in contrast, achieved a form of entanglement between two photons that is more immediately useful, although their connection was shorter lived. The teams described their work in two studies published June 2 in the journal Nature.
These results "provide key important steps forward on building blocks of future quantum repeater chains," Ronald Hanson, a quantum communications researcher at the Delft University of Technology in the Netherlands, told Live Science in an email. "For the field working on solid-state ensemble-based memories, these push the state of the art significantly."
A long road ahead
The ICFO experiment represents the culmination of a decade of work spearheaded by physicist Hugues de Riedmatten to develop the procedures, materials and devices needed to create the heralded link. Grandi and his ICFO colleague Dario Lago-Rivera also went to extreme lengths to isolate the rudimentary repeater's components from the turmoil of the world. If vibrations from the building or a blast of hot air caused the meters-long cable to stretch by even a dozen nanometers, for instance, the disturbance would ruin the experiment.
Despite the progress, practical quantum repeaters that can reliably entangle memories across cities — much less continents — remain years away. The ICFO memories can remember their qubits for only 25 microseconds, enough time to entangle with another memory no farther than 3 miles (5 kilometers) away. The finicky system is also unreliable, with attempts to write a photon to memory succeeding just 25% of the time.
Nevertheless, the researchers have various ideas for how to improve their setup. Buoyed by the success of combining so many quantum elements, they believe they're on the path to stretching entanglement and quantum communications from neighboring labs to neighboring cities.
"This was a proof-of-principle starting point," Grandi said. We just wanted to "see if everything works."
Originally published on Live Science.
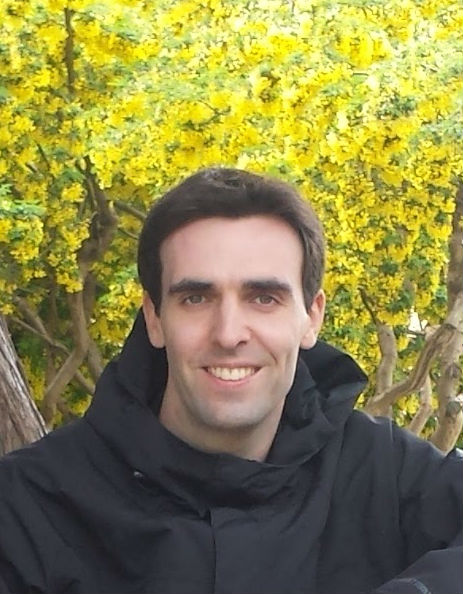
Charlie Wood is a staff writer at Quanta Magazine, where he covers physics both on and off the planet. In addition to Live Science, his work has also appeared in Popular Science, Scientific American, The Christian Science Monitor, and other publications. Previously, he taught physics and English in Mozambique and Japan, and he holds an undergraduate degree in physics from Brown University.