New study turns our understanding of ice upside down
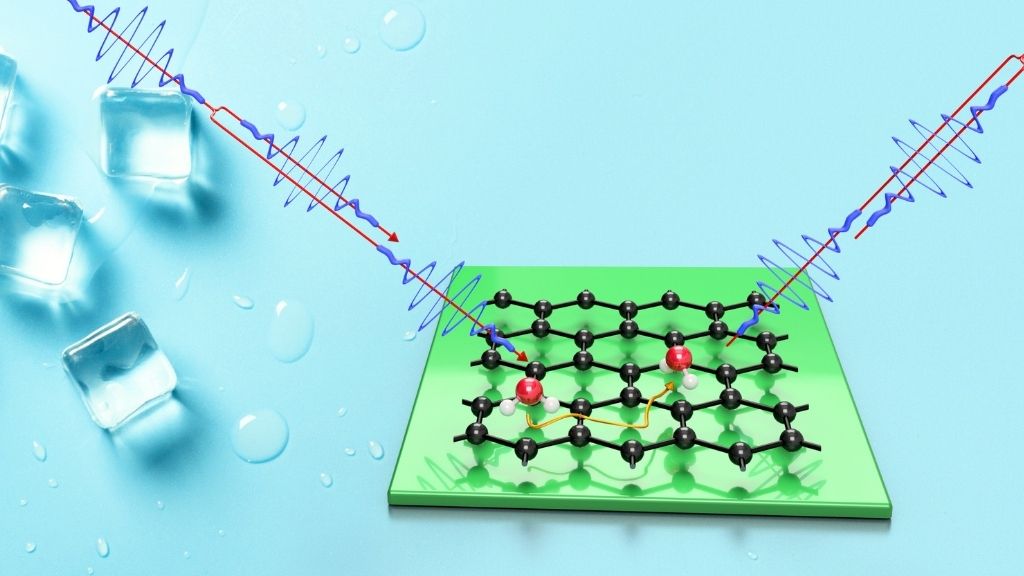
As water freezes into ice, free-wheeling water molecules suddenly stop moving and begin forming ice crystals with their neighbors — but ironically, they need a bit of heat to do so, scientists recently discovered.
Yes, you read that right: You actually need some extra heat to freeze water into ice. That's according to a new study, published Tuesday (May 25) in the journal Nature Communications, which zoomed in on the movement of individual water molecules deposited on a frigid graphene surface. The research team used a technique called helium spin-echo, first developed at the University of Cambridge, which involves firing a beam of helium atoms at the water molecules, and then tracking how those helium atoms scatter once they ram into the forming ice.
The technique works similarly to radar detectors that use radio waves to determine how quickly a car is zipping down the highway, said first author Anton Tamtögl, a postdoctoral researcher at the Institute of Experimental Physics at Graz University of Technology in Austria. "This is more like a radar trap for molecules, on an atomic scale," he told Live Science.
Related: The 18 biggest unsolved mysteries in physics
The method not only enabled the researchers to collect data from each teensy atom in their experiments, but also helped them record the earliest stage of ice formation, known as "nucleation," when water molecules first begin to coalesce into ice. Nucleation takes place at mind-boggling speeds — within a fraction of a billionth of a second — and as a result, many studies of ice formation focus on the period of time just after nucleation, when patches of ice have already formed and begin to merge into a kind of thick film, Tamtögl said.
For instance, studies that rely on conventional microscopes can't capture what occurs at the start of nucleation, because the instruments aren’t capable of snapping images fast enough to keep up with the speedy water molecules, he said. Scientists sometimes slow down this molecular movement by applying liquid nitrogen to their experiments, lowering the temperature to around minus 418 degrees Fahrenheit (minus 250 degrees Celsius), but if you want to observe ice freezing at warmer temperatures, "then you need to use this spin-echo," Tamtögl said. In their own experiments, the team cooled the graphene surface to between minus 279 F and minus 225 F (minus 173 C to minus 143 C).
But when the team applied helium spin-echo to water molecules deposited on the graphene, they discovered something counterintuitive.
Sign up for the Live Science daily newsletter now
Get the world’s most fascinating discoveries delivered straight to your inbox.
"What came as a surprise to us is this signature we had from the repulsive interaction — from the water molecules 'not liking each other,'" Tamtögl said. Essentially, as the team put water down upon the graphene surface, the molecules appeared to repel each other at first, maintaining a degree of distance.
"They had to kind of overcome this barrier before they could form the islands" of ice upon the graphene surface, he said. To better understand the nature of this repulsive force, and how the molecules overcame it, the team generated computational models to map out the interactions of the water molecules in different configurations.
Related: What's that? Your physics questions answered
These models revealed that, upon being placed on cold graphene, the water molecules all orient in the same direction, with their two hydrogen atoms pointed down; the hydrogen atoms in a water molecule stick off from the central oxygen atom like two mouse ears. These water molecules somewhat cluster together on the surface of the graphene, but due to their orientation, a few molecules’ worth of empty space still persists between them.
To bond into ice crystals, the molecules must scooch a tiny bit closer to one another and break out of their uniform orientation. "That's what forms this barrier, where it will cost energy" to nucleate, Tamtögl said.
By adding more energy to the system in the form of heat, the team found they could nudge the water molecules toward each other and allow them to reorient and nucleate, finally forming ice. Adding more water molecules to the system also helped overcome the energy barrier, as the system became more and more crowded and molecules cozied up to one another, Tamtögl said.
All these interactions take place on incredibly short timescales, so this brief struggle to overcome the energy barrier passes in a flash.
Tamtögl and his colleagues plan to study whether ice nucleation unfolds similarly on different surfaces. For instance, so-called "white graphene," also known as hexagonal boron nitride, shares a similar structure to normal graphene but forms stronger bonds with water molecules, so nucleation may unfold more slowly on that type of surface, he said.
More broadly, learning exactly how ice forms would be useful in many scientific applications. For instance, with fine-grain knowledge of ice formation, scientists could potentially improve technologies meant to prevent aeronautical equipment, wind turbines and communication towers from icing over, the authors wrote in their paper. Ice appears on cosmic dust grains and in Earth's atmosphere, and of course in glaciers; so unpacking the nitty-gritty physics of ice could have far-reaching relevancy in research.
"Water is such a ubiquitous molecule, right? But it appears there's still so much we don't understand in detail, even though it's a simple molecule," Tamtögl said. "There's still much more to be learned."
Originally published on Live Science.
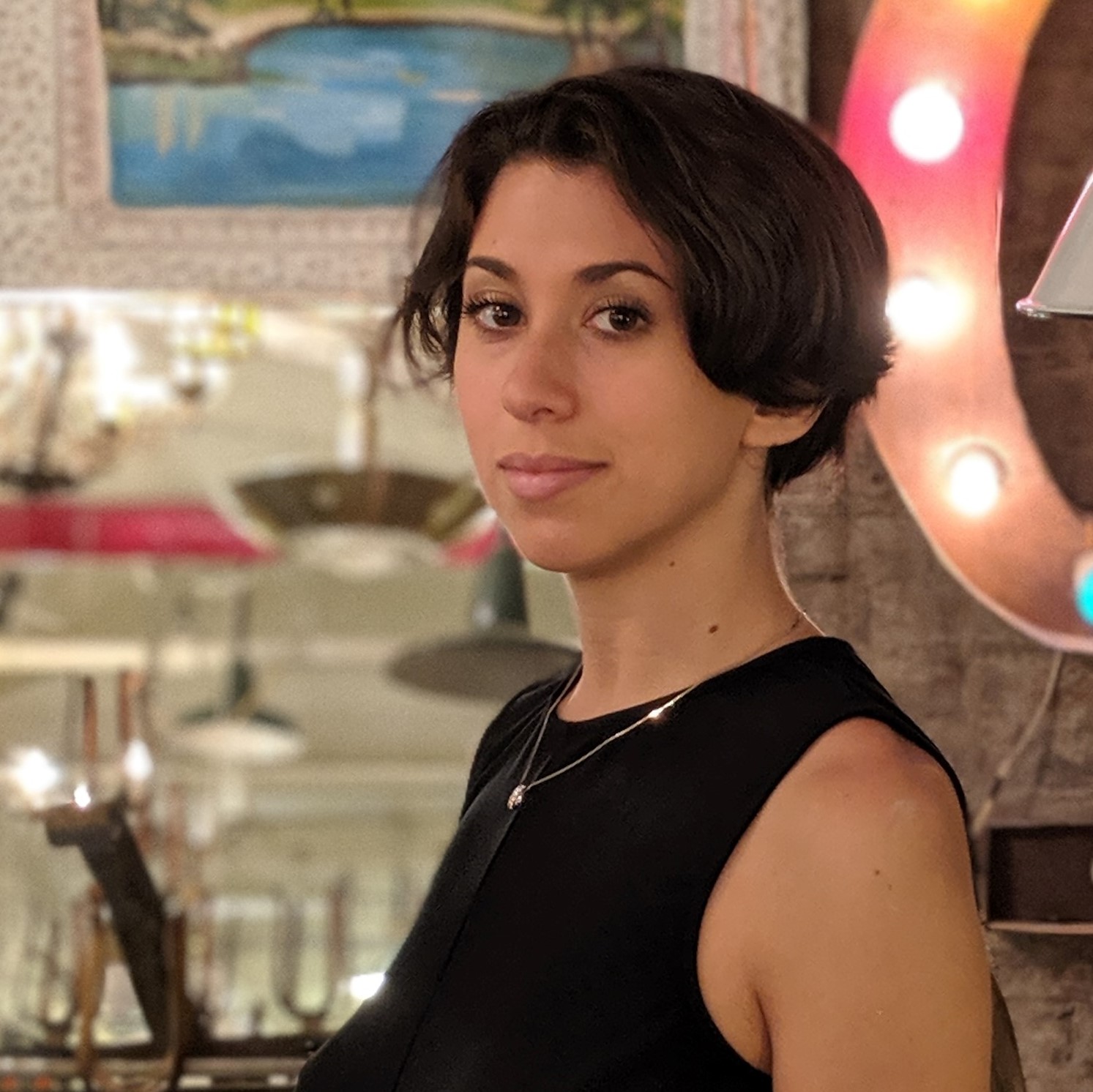
Nicoletta Lanese is the health channel editor at Live Science and was previously a news editor and staff writer at the site. She holds a graduate certificate in science communication from UC Santa Cruz and degrees in neuroscience and dance from the University of Florida. Her work has appeared in The Scientist, Science News, the Mercury News, Mongabay and Stanford Medicine Magazine, among other outlets. Based in NYC, she also remains heavily involved in dance and performs in local choreographers' work.