
Weird 'gravitational molecules' could orbit black holes like electrons swirling around atoms
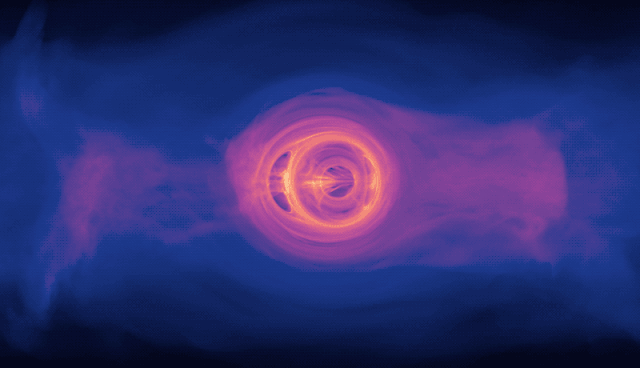
Black holes are notable for many things, especially their simplicity. They're just … holes. That are "black." This simplicity allows us to draw surprising parallels between black holes and other branches of physics. For example, a team of researchers has shown that a special kind of particle can exist around a pair of black holes in a similar way as an electron can exist around a pair of hydrogen atoms — the first example of a "gravitational molecule." This strange object may give us hints to the identity of dark matter and the ultimate nature of space-time.
Ploughing the field
To understand how the new research, which was published in September to the preprint database arXiv, explains the existence of a gravitational molecule, we first need to explore one of the most fundamental –- and yet sadly almost never talked about –- aspects of modern physics: the field.
Related: The 12 strangest objects in the universe
A field is a mathematical tool that tells you what you might expect to find as you travel from place to place in the universe. For example, if you've ever seen a TV weather report of temperatures in your local area, you're looking at a viewer-friendly representation of a field: As you travel around your town or state, you'll know what kind of temperatures you're likely to find, and where (and whether you need to bring a jacket).
This kind of field is known as a "scalar" field, because "scalar" is the fancy mathematical way of saying "just a single number." There are other kinds of fields out there in physics-land, like "vector" fields and "tensor" fields, which provide more than one number for every location in space-time. (For example, if you see a map of wind speed and direction splashed on your screen, you're looking at a vector field.) But for the purposes of this research paper, we only need to know about the scalar kind.
The atomic power couple
In the heydays of the mid-20th century, physicists took the concept of the field — which had been around for centuries at that point, and was absolutely old-hat to the mathematicians — and went to town with it.
They realized that fields aren't just handy mathematical gimmicks — they actually describe something super-fundamental about the inner workings of reality. They discovered, basically, that everything in the universe is really a field.
Sign up for the Live Science daily newsletter now
Get the world’s most fascinating discoveries delivered straight to your inbox.
Related: The 11 most beautiful mathematical equations
Take the humble electron. We know from quantum mechanics that it's pretty tough to pin down exactly where an electron is at any given moment . When quantum mechanics first emerged, this was a pretty nasty mess to understand and untangle, until the field came along.
In modern physics, we represent the electron as a field — a mathematical object that tells us where we're likely to spot the electron the next time we look. This field reacts to the world around it — say, because of the electric influence of a nearby atomic nucleus — and modifies itself to change where we ought to see the electron.
The end result is that electrons can appear only in certain regions around an atomic nucleus, giving rise to the entire field of chemistry (I'm simplifying a bit, but you get my point).
Black hole buddies
And now the black hole part. In atomic physics, you can completely describe an elementary particle (like an electron) in terms of three numbers: its mass, its spin and its electric charge. And in gravitational physics, you can completely describe a black hole in terms of three numbers: its mass, its spin and its electron charge.
Coincidence? The jury's out on that one, but for the time being we can exploit that similarity to better understand black holes.
In the jargon-filled language of particle physics that we just explored, you can describe an atom as a tiny nucleus surrounded by the electron field. That electron field responds to the presence of the nucleus, and allows the electron to appear only in certain regions. The same is true for electrons around two nuclei, for example in a diatomic molecule like hydrogen (H2.)
You can describe the environment of a black hole similarly. Imagine the tiny singularity at a black heart somewhat akin to the nucleus of an atom, while the surrounding environment — a generic scalar field — is similar to the one that describes a subatomic particle. That scalar field responds to the presence of the black hole, and allows its corresponding particle to appear only in certain regions. And just as in diatomic molecules, you can also describe scalar fields around two black holes, like in a binary black hole system.
The authors of the study found that scalar fields can indeed exist around binary black holes. What's more, they can form themselves into certain patterns that resemble how electron fields arrange themselves in molecules. So, the behavior of scalar fields in that scenario mimics how electrons behave in diatomic molecules, hence the moniker "gravitational molecules."
Why the interest in scalar fields? Well for one, we don't understand the nature of dark matter or dark energy, and it's possible both dark energy and dark matter could be made up of one or more scalar fields), just like electrons are made up of the electron field.
If dark matter is indeed composed of some sort of scalar field, then this result means that dark matter would exist in a very strange state around binary black holes — the mysterious dark particles would have to exist in very specific orbits, just like electrons do in atoms. But binary black holes don't last forever; they emit gravitational radiation and eventually collide and coalesce into a single black hole. These dark matter scalar fields would affect any gravitational waves emitted during such collisions, because they would filter, deflect and reshape any waves passing through regions of increased dark matter density. This means we might be able to detect this kind of dark matter with enough sensitivity in existing gravitational wave detectors.
In short: We soon might be able to confirm the existence of gravitational molecules, and through that open a window into the hidden dark sector of our cosmos.
Originally published on Live Science.
Paul M. Sutter is a research professor in astrophysics at SUNY Stony Brook University and the Flatiron Institute in New York City. He regularly appears on TV and podcasts, including "Ask a Spaceman." He is the author of two books, "Your Place in the Universe" and "How to Die in Space," and is a regular contributor to Space.com, Live Science, and more. Paul received his PhD in Physics from the University of Illinois at Urbana-Champaign in 2011, and spent three years at the Paris Institute of Astrophysics, followed by a research fellowship in Trieste, Italy.