Ghost in the Machine: Atom Smasher's 'New Particle' Was Illusion
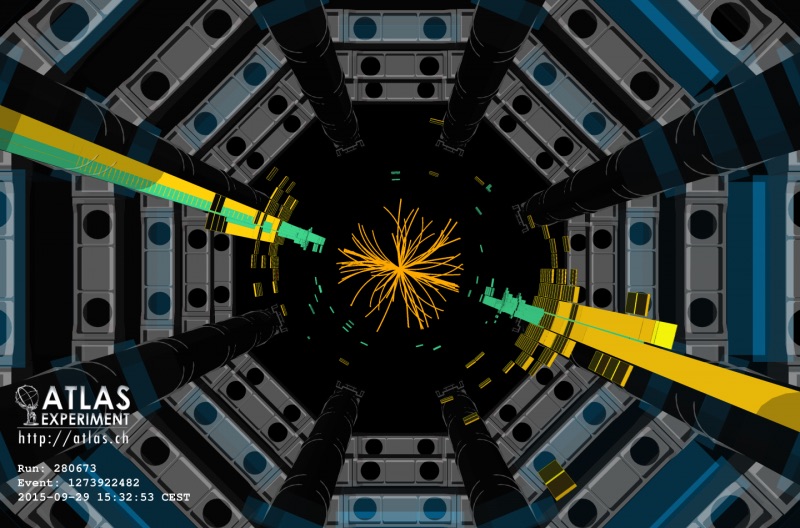
When you're searching high and low for your lost keys, sometimes the places where you don't see them can help you narrow down where they might be. In science, the search for new physics often takes a similar path.
In December 2015, scientists at the Large Hadron Collider (LHC) — the world's largest particle accelerator — thought they may have seen a hint of a brand-new particle, and with it, a window into physics beyond what scientists know now. But the findings turned out to be ghosts, a statistical fluke.
Yet despite the negative result, the fact that there is nothing there shows that reigning theories of particle physics are working remarkably well, experts said. But that result only deepens the mysteries physicists are trying to solve, and pushes them to find out just where new particles or forces could be hiding. [The 9 Biggest Unsolved Mysteries in Physics]
"The bad news is [the measurements] don't show anything," said theoretical physicist Matt Strassler. "The good news is that it did a really good job of not showing anything."
Strassler himself recently gave a talk at the European Organization for Nuclear Research (CERN), which runs the LHC, on what can be learned from such results, or lack thereof. "It's like if you squint trying to see something — sometimes you'll see something, and sometimes it will be an illusion," Strassler told Live Science.
High-energy collisions
The LHC uses about 9,600 massive magnets to circulate streams of protons within a 17-mile-long (27 kilometers) ring. These protons are accelerated to near the speed of light and then smashed together. The resulting collisions unleash cascades of subatomic particles and radiation that can provide clues about the building blocks of matter.
After a two-year hiatus for upgrades, the LHC was fired up again last year, this time running at higher energies.
Sign up for the Live Science daily newsletter now
Get the world’s most fascinating discoveries delivered straight to your inbox.
What scientists found in December was that two detection systems, one called ATLAS and the other called CMS, found a curious bump in the plots of energy versus "events." (Events are essentially detections of photons or particles.)
The bump was large enough that it looked interesting to scientists. If real, it could have been evidence of a particle nobody has seen before at energies of 750 billion electron volts (GeV). During the LHC's current run, it can reach energies of almost 13 trillion electron volts (TeV).
Data from the debris
When smashed together, the energy the protons carry will turn into particles, each with a characteristic energy. Most of these particles are short-lived, though, and decay into other particles and photons.
For this reason, particle detections are often indirect. This is what happened in 2012, when scientists discovered the Higgs boson, the elementary particle that is thought to explain how other particles get their mass. And this is why the most recent bump was so intriguing.
But now, new data from CMS, collected since December, shows that the 750 GeV bump was likely an illusion — a statistical artifact of the kind that sometimes crops up in experiments like this, said Michael Peskin, a theoretical physicist at the SLAC National Accelerator Laboratory. [Beyond Higgs: 5 Elusive Particles That May Lurk in the Universe]
Even back in December, some physicists — Peskin among them — had doubts. He noted the teams working on the LHC issued a statement that said, effectively, they weren't issuing one. "The statement said the statistical significance was too low to report an observation," Peskin said.
But that doesn't mean it's a useless result, Strassler said. Nor does it mean the spate of papers theorizing about what the observation could be are just wrong and not worthy of consideration, he added. Such work can often yield important insights down the road.
"This process of being sure nothing has been missed is going to take longer than discovering something," he said. "Sometimes things at 750 GeV might be relevant for a particle 10 times smaller that hasn't been discovered yet."
On the bright side
Negative results like this are also important because they show just where the conceptual problems with current theories might lie. In this case, the theory is the Standard Model, the reigning theory in physics that describes the bevy of subatomic particles that make up the universe. [Wacky Physics: The Coolest Little Particles in Nature]
But phenomena such as dark matter, the invisible stuff that is thought to make up 85 percent of the matter in the universe, suggest that the Standard Model isn't complete. And physicists and cosmologists have a difficult time explaining why the universe is dominated by matter rather than antimatter, or why the Higgs field that gives things mass is the strength that it is.
"For the Higgs boson, we end up having to say, 'That's the way it is,'" Peskin said. "And I don't like having to say that." There has to be a reason, he added, that the Higgs field looks the way it does, and the Standard Model by itself doesn't really provide the answer.
This is where negative results can be useful. For example, there are many extensions to the Standard Model that propose something called supersymmetry, or SUSY. These theories say that every particle has a yet-to-be-discovered partner particle. The existence of such partners would help physicists understand why the Higgs boson has the value it does (and it predicts that there is a supersymmetric partner to the Higgs, as well).
Over the years, negative results have helped narrow down the SUSY models that work, Peskin said. "Basically every SUSY model on the table in the mid-2000s is now excluded," he said. This doesn't mean SUSY is wrong, but it helps researchers focus the theoretical work.
Lessons from history
The history of science records a number of negative results that led to greater insights. In 1887, Albert Michelson and Edward Morley conducted an experiment to find the aether, a proposed medium that was thought to carry light waves. If the aether existed, the speed of light should have changed depending on the direction of the beam. It didn't, and years later, Albert Einstein used that negative result as part of the formulation of his theory of relativity, which says it is space-time itself that changes in order to keep the speed of light the same in all reference frames. [8 Ways You Can See Einstein's Theory of Relativity in Real Life]
The issue was the way people conceived of light waves. "Maybe [light] waves were different from other waves we knew about," Strassler said, in that they didn't need a medium to travel through.
Strassler noted that nobody has made this kind of fundamental leap yet. In the case of Michelson-Morley, for instance, it was realizing that light waves don't need a medium. For particle physicists, it's not even clear what the insight has to be. It's possible the problem is technical and scientists just need better accelerators and detectors to find new particles. Or it could be conceptual, as it was for Michelson and Morley, Strassler said.
Some physicists say the negative result likely won't have any significance beyond just being a statistical ghost. "We know that the Standard Model is not a complete theory, and that it has to be extended at some energy scale. But, theoretically, there are millions of possible extensions, and we need a clue from experiment which is the correct one," said Adam Falkowski, a theoretical particle physicist at the Centre National de la Recherche Scientifique (CNRS) in Paris, and co-author of a paper describing the implications of the 750 GeV bump being a real effect.
Still, the result could help in some ways, said Nhan Tran, a postdoctoral research fellow at the Fermi National Accelerator Laboratory in Illinois."It contributes to our knowledge of what's not there," he said. "Helping us focus on where we should be looking."
For his part, Peskin said he thinks the comparison between current experiments at the LHC and early work to find the proposed aether is a bit overstated. "Michelson [and] Morely blew away the previous theory," he said. The Standard Model is on firmer ground, he said. However, he added that results like this one make him less confident about ideas like SUSY. "On Tuesdays, Thursdays and Saturdays, I believe in it," he said. "On other days, I think it could be anything."
At the same time, the LHC is a powerful new tool, Peskin said. "Our ability to detect SUSY particles is a lot more powerful," he said. "If you believe they are there, it might turn up in the next year."
Original article on Live Science.
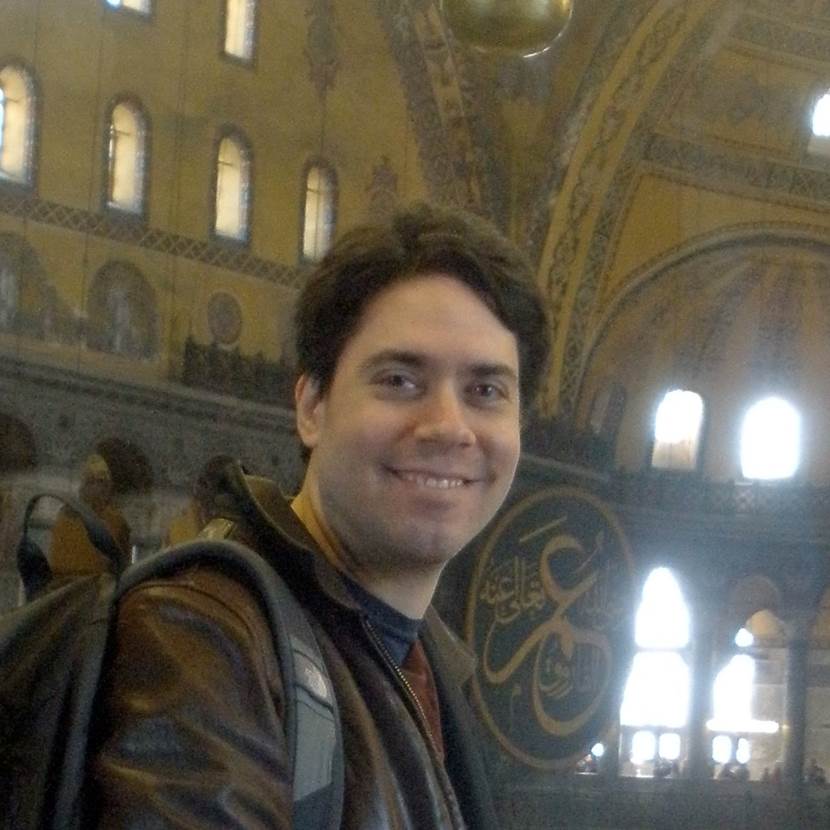