How Do You Weigh an Atom?
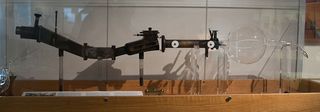
Update: This article was updated on Sept. 11, 2017 by Rachel Ross, Live Science Contributor.
Imagine plopping an atom down on a scale. As you do so, skin cells that are trillions of atoms thick flake off your hand and flutter down all around it, burying it in a pile of atomic doppelgangers. Meanwhile, moisture and atmospheric particles shoot about, bouncing on and off the scale and sending its atom-sensitive needle whipping back and forth like a windshield wiper. And by the way, how did you manage to isolate a single atom in the first place?
A moment's thought shows you can't weigh an atom on a traditional scale.
Instead, physicists for over a century have used an instrument called a mass spectrometer. Invented in 1912 by physicist J.J. Thomson and improved incrementally, it works like this: First, physicists "ionize" a gas of atoms by firing a beam of particles at the gas, which either adds electrons to the atoms in it or knocks a few of their electrons off, depending on the type of particle beam used. This gives the atoms — now known as "ions" — a net negative or positive electric charge.
Next, the ions are sent through a tube in which they're subjected to electric and magnetic fields. Both of these fields exert a force on the ions, and the strengths of the two forces are proportional to the ions' charge (neutral atoms don't feel the forces). The electric force causes the ions to change speed, while the magnetic force bends their path.
The ions are then collected by "Faraday cups" at the end of the tube, generating a current in wires attached to the cups. By measuring where and when the stream of ions hits the Faraday cups, the physicists can determine how much they must have accelerated, and in what direction, as a result of the electric and magnetic forces. Lastly, by way of Newton's second law of motion, F=ma, rearranged as m=F/a, the physicists divide the total force acting on the ions by their resulting acceleration to determine the ions' mass.
The mass of the electron has also been determined using a mass spectrometer — in that case, electrons were simply sent through the instrument themselves. That measurement enables physicists to determine the mass of an atom when it has the correct number of electrons, rather than a dearth or surplus of them.
Sign up for the Live Science daily newsletter now
Get the world’s most fascinating discoveries delivered straight to your inbox.
Using a mass spectrometer, physicists have determined the mass of a hydrogen atom to be 1.660538921(73) × 10-27 kilograms, where the parenthetical digits are not known with complete certainty. That's accurate enough for most purposes.
Good vibrations
Another way that the mass of an atom can be found is by measuring its vibration frequency and solving backwards, according to Jon R. Pratt’s 2014 article in the Journal of Measurement Science.
The vibration of an atom can be determined in a few ways, including atom interferometry, in which atomic waves are coherently split and later recombined, according to Alex Cronin, an associate professor in the department of physics at the University of Arizona; and frequency combs, which use spectrometry to measure vibrations. The frequency can then be used with the Planck constant to find the energy of the atom (E = hv, where h is the Planck constant and v is the frequency). The energy can then be used with Einstein's famous equation, E = mc2, to solve for the mass of the atom when it is rearranged to m = E/c2.
A third way to measure the mass of an atom is described in a 2012 article published in Nature Nanotechnology by J. Chaste, et al. This method involves using carbon nanotubes at low temperatures and in a vacuum and measuring how the vibration frequency changes depending on the mass of the particles attached to them. This scale can measure masses down to one yoctogram, less than the mass of a single proton (1.67 yoctograms).
The test was with a 150-nanometer carbon nanotube suspended over a trench. The nanotube was plucked like a guitar string, and this produced a natural vibration frequency that was then compared to the vibration patterns when the nanotube came into contact with other particles. The amount of mass that is on the nanotube will change the frequency that is produced.
Ye olde mass
What about before the days of mass spectrometers, when chemists were fuzzy about what an atom even was? Then, they primarily measured the weights of the atoms that composed various elements in terms of their relative masses, rather than their actual masses. In 1811, the Italian scientist Amedeo Avogadro realized that the volume of a gas (at a given pressure and temperature) is proportional to the number of atoms or molecules composing it, regardless of which gas it was. This useful fact allowed chemists to compare the relative weights of equal volumes of different gases to determine the relative masses of the atoms composing them.
They measured atomic weights in terms of atomic mass units (amu), where 1 amu was equal to one-twelfth of the mass of a carbon-12 atom. When in the second half of the 19th century, chemists used other means to approximate the number of atoms in a given volume of gas — that famous constant known as Avogadro's number — they began producing rough estimates of the mass of a single atom by weighing the volume of the whole gas, and dividing by the number.
The Difference Between Atomic Weight, Mass and Number
Many people use the terms weight and mass interchangeably, and even most scales offer options in units such as pounds and kilograms. And while mass and weight are related, they are not the same thing. When discussing atoms, many people use atomic weight and atomic mass interchangeably, even though they aren't quite the same thing either.
Atomic mass is defined as the number of protons and neutrons in an atom, where each proton and neutron has a mass of approximately 1 amu (1.0073 and 1.0087, respectively). The electrons within an atom are so miniscule compared to protons and neutrons that their mass is negligible. The carbon-12 atom, which is still used as the standard today, contains six protons and six neutrons for an atomic mass of twelve amu. Different isotopes of the same element (same element with different amounts of neutrons) do not have the same atomic mass. Carbon-13 has an atomic mass of 13 amu.
Atomic weight, unlike the weight of an object, has nothing to do with the pull of gravity. It is a unitless value that is a ratio of the atomic masses of naturally occurring isotopes of an element compared with that of one-twelfth the mass of carbon-12. For elements such as beryllium or fluorine that only have one naturally occurring isotope, the atomic mass is equal to the atomic weight.
Carbon has two naturally occurring isotopes – carbon-12 and carbon-13. The atomic masses of each are 12.0000 and 13.0034, respectively, and knowing their abundances in nature (98.89 and 1.110 percent, respectively), the atomic weight of carbon is calculated to be about 12.01. The atomic weight is very similar to the mass of carbon-12 due to the majority of carbon in nature being made of the carbon-12 isotope.
The atomic weight of any atom can be found by multiplying the abundance of an isotope of an element by the atomic mass of the element and then adding the results together. This equation can be used with elements with two or more isotopes:
- Carbon-12: 0.9889 x 12.0000 = 11.8668
- Carbon-13: 0.0111 x 13.0034 = 0.1443
- 11.8668 + 0.1443 = 12.0111 = atomic weight of carbon
And there is still a third value that is used when discussing measurements related to atoms: atomic number. The atomic number is defined by the number of protons in an element. An element is defined by the number of protons the nucleus contains and doesn't have anything to do with how many isotopes the element has. Carbon always has an atomic number of 6 and uranium always has an atomic number of 92.
Additional reporting by Rachel Ross, Live Science Contributor.
Additional resources
Natalie Wolchover was a staff writer for Live Science from 2010 to 2012 and is currently a senior physics writer and editor for Quanta Magazine. She holds a bachelor's degree in physics from Tufts University and has studied physics at the University of California, Berkeley. Along with the staff of Quanta, Wolchover won the 2022 Pulitzer Prize for explanatory writing for her work on the building of the James Webb Space Telescope. Her work has also appeared in the The Best American Science and Nature Writing and The Best Writing on Mathematics, Nature, The New Yorker and Popular Science. She was the 2016 winner of the Evert Clark/Seth Payne Award, an annual prize for young science journalists, as well as the winner of the 2017 Science Communication Award for the American Institute of Physics.