Stephen Hawking's most famous prediction could mean that everything in the universe is doomed to evaporate, new study says
A new theory has radically revised Stephen Hawking's 1974 theory of black holes to predict that all objects with mass may eventually disappear.
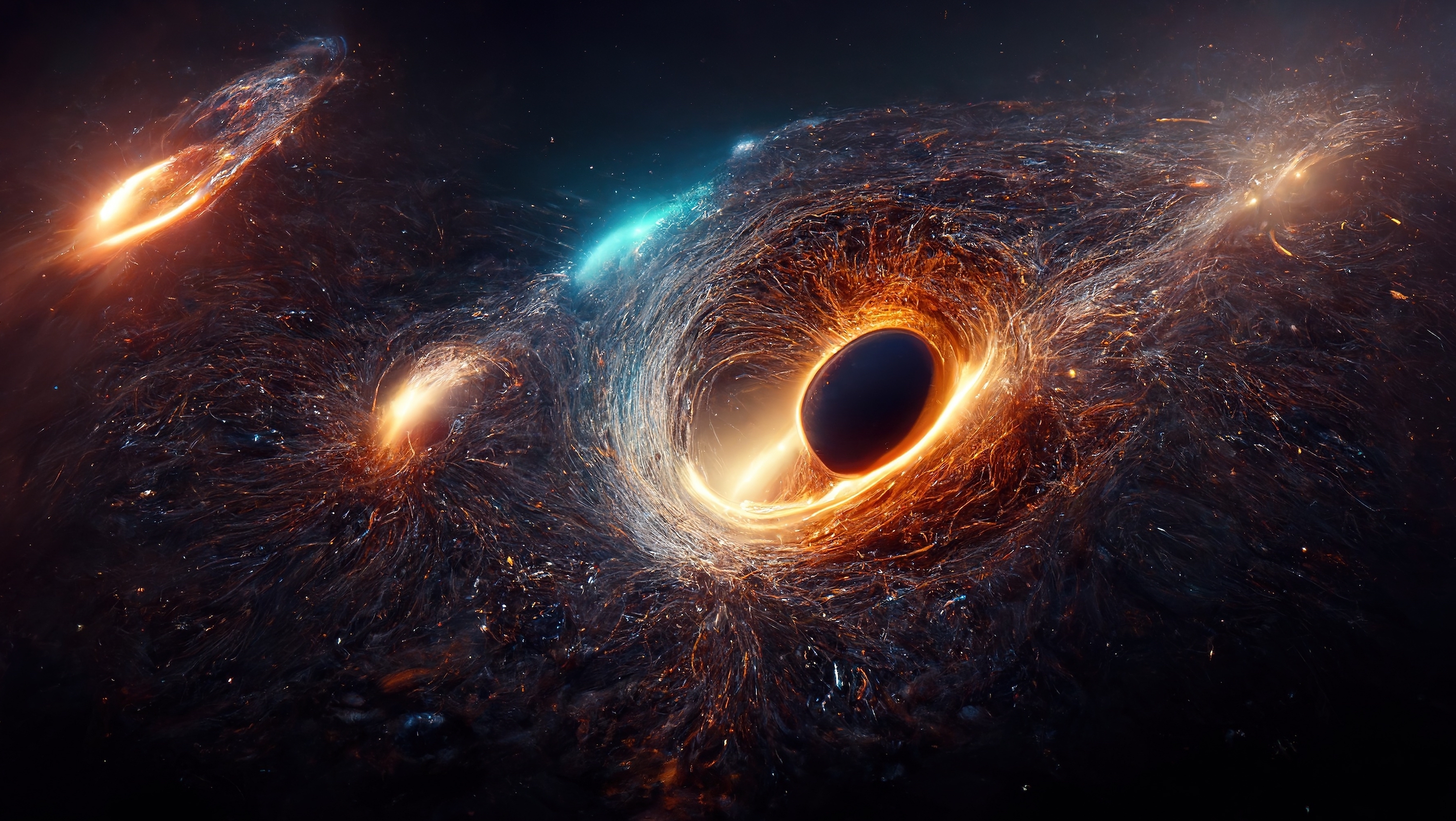
Stephen Hawking's most famous theory about black holes has just been given a sinister update — one that proclaims that everything in the universe is doomed to evaporate.
In 1974, Hawking proposed that black holes eventually evaporate by losing what's now known as Hawking radiation — a gradual draining of energy in the form of light particles that spring up around black holes' immensely powerful gravitational fields. Now, a new update to the theory has suggested that Hawking radiation isn't just created by stealing energy from black holes, but from all objects with enough mass.
If the theory is true, it means that everything in the universe will eventually disappear, its energy slowly bled from it in the form of light.
Related: Lab-grown black hole may prove Stephen Hawking's most challenging theory right
"That means that objects without an event horizon [the gravitational point of no return beyond which nothing, not even light, can escape a black hole], such as the remnants of dead stars and other large objects in the universe, also have this sort of radiation," lead author Heino Falcke, a professor of astrophysics at Radboud University in the Netherlands, said in a statement. "And, after a very long period, that would lead to everything in the universe eventually evaporating, just like black holes. This changes not only our understanding of Hawking radiation but also our view of the universe and its future."
The researchers published their findings June 2 in the journal Physical Review Letters.
Space-time monsters
According to quantum field theory, there is no such thing as an empty vacuum. Space is instead teeming with tiny vibrations that, if imbued with enough energy, randomly burst into virtual particles, producing very-low-energy packets of light, or photons.
Sign up for the Live Science daily newsletter now
Get the world’s most fascinating discoveries delivered straight to your inbox.
In a landmark paper published in 1974, Hawking famously predicted that the extreme gravitational force felt at the mouths of black holes — their event horizons — would summon photons into existence in this way. Gravity, according to Einstein's theory of general relativity, distorts space-time, so that quantum fields get more warped the closer they get to the immense gravitational tug of a black hole's singularity.
Because of the uncertainty and weirdness of quantum mechanics, Hawking said this warping creates uneven pockets of differently moving time and subsequent spikes of energy across the field. These energy mismatches make photons appear in the contorted space around black holes, siphoning energy from the black hole's field so they can burst into existence. If the particles then escape the black hole, this energy theft led Hawking to conclude that — over a vast timescale much longer than the current age of the universe — black holes would eventually lose all of their energy and disappear completely.
But if a gravitational field is all that's needed to produce quantum fluctuations and photons, what's stopping any object with a space-time warping mass from creating Hawking radiation? Does Hawking radiation need the special condition of a black hole's event horizon, or can it be produced anywhere in space? To probe these questions, the authors of the new study analyzed Hawking radiation through the lens of a long-predicted process called the Schwinger effect, in which matter can theoretically be generated from the powerful distortions caused by an electromagnetic field.
Sure enough, by applying the framework of the Schwinger effect to Hawking's theory, the theoretical physicists produced a mathematical model that reproduced Hawking radiation in spaces experiencing a range of gravitational field strengths. According to their new theory, an event horizon isn't necessary for energy to slowly leak from a massive object in the form of light; the object's gravitational field is good enough on its own.
"We show that far beyond a black hole the curvature of space-time plays a big role in creating radiation," second author Walter van Suijlekom, a professor of mathematics at Radboud University, said in the statement. "The particles are already separated there [beyond the black hole] by the tidal forces of the gravitational field."
What the researchers' theory means in reality isn't clear. Possibly, as the matter that makes up stars, neutron stars, and planets ages, it will eventually undergo an energy transition into a completely new ultralow energy state. This might be enough to eventually collapse all matter into black holes, which could continue to slowly drip out light until they too disappear without a trace.
Unfortunately (or fortunately, depending on any misgivings you may have about evaporating), all of this is just speculation awaiting confirmation. To figure out if it's a true prediction of our universe's eventual fate, physicists will need to spot some Hawking radiation being produced around gravitationally dense objects — both around black holes and planets, stars, or neutron stars. If everything is destined to disappear in a flash of cool light, there should be plenty of places to look.
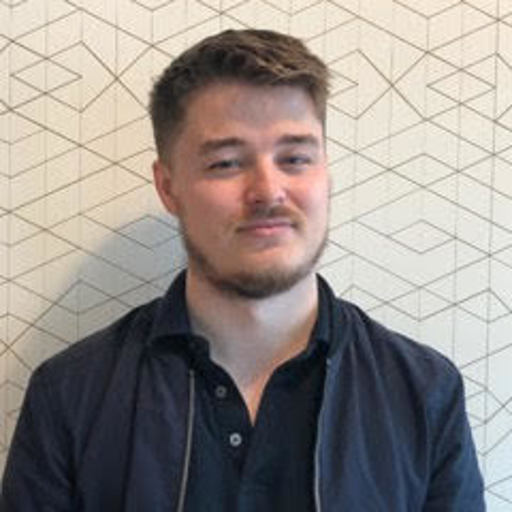
Ben Turner is a U.K. based staff writer at Live Science. He covers physics and astronomy, among other topics like tech and climate change. He graduated from University College London with a degree in particle physics before training as a journalist. When he's not writing, Ben enjoys reading literature, playing the guitar and embarrassing himself with chess.