Major CERN experiment proves antigravity doesn't exist — at least when it comes to antimatter
The new research, which shows that elusive antimatter falls downward toward the Earth rather than levitating, proves Albert Einstein right yet again.
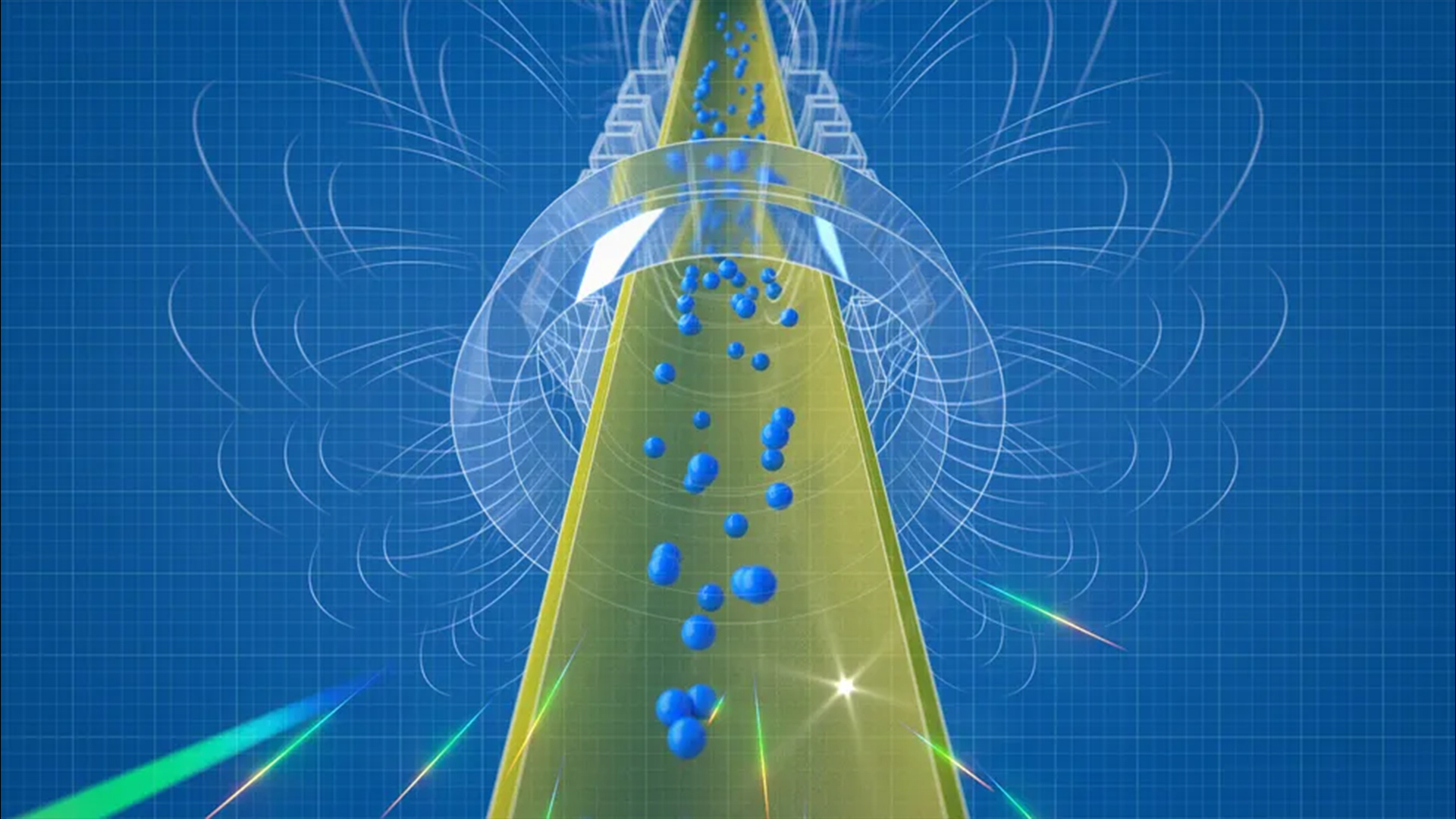
On Sept. 27, an international team of physicists reported a major finding about an elusive form of matter known as antimatter. It appears that antimatter responds to gravity the same way regular matter does, validating theories proposed by Albert Einstein more than a century ago.
This result marks the first-ever direct observation of free-falling antimatter, in which atoms are made of antiprotons instead of protons and antielectrons (positrons) instead of electrons. Antiprotons are basically negatively charged protons (protons are positive in normal matter atoms) and positrons are positively charged electrons (electrons are negative in normal matter atoms).
The new research ultimately proved that atomic antihydrogen — made up of one antiproton in the center with a positively charged positron orbiting around it — is pulled downward due to gravity instead of upward like you might expect with a form of matter that presents as the "opposite" of normal matter.
Furthermore, close to three decades after antihydrogen was first created in a lab, today's scientific triumph is yet another confirmation of Einstein's general theory of relativity, which predicts that all masses, irrespective of differences in their internal structures, react to gravity in a similar manner.
"If you walk down the halls of this department and ask the physicists, they would all say that this result is not the least bit surprising. That's the reality," Jonathan Wurtele, a physics professor at the University of California at Berkeley who first proposed the experiment over a decade ago and a co-author of the new study, said in a statement. "But most of them will also say that the experiment had to be done because you never can be sure."
Related: Antimatter Is Both a Particle and a Wave, New Experiment Confirms
Capturing the miniscule
Wurtele and his team created, trapped and studied antihydrogen particles at The European Center for Nuclear Research (better known by its French acronym, CERN). The particles were trapped within what was essentially a magnetic bottle, both ends of which contained controllable magnetic fields. To witness the effects of gravity on the antihydrogen particles, researchers reduced the magnetic field strength at each end to let the particles escape.
Get the world’s most fascinating discoveries delivered straight to your inbox.
When each particle wandered to the top or bottom of the magnetic bottle, it zapped in a flash. Researchers then counted those flashes and found a higher number wandered to the bottom of the bottle compared to the top. A staggering 80% of them behaved in such a way, in fact, and this result held true for a dozen repeats of the experiment. According to the new study, that conclusively demonstrated that gravity causes the antihydrogen to fall downward.
"This gives us a powerful experimental knob that allows us, basically, to believe the experiment actually worked because we can prove to ourselves that we can control the experiment in a predictable manner," Joel Fajans, a physics professor at UC Berkeley and a co-author of the new study, said in the statement.
The team also found that the gravitational acceleration of antihydrogen was close to that of normal matter, which is 9.8 meters (32 feet) per second squared. That result is expected to hold true for other antimatter particles too, researchers say.
"It would be doubly surprising if this was not true (first, that something fell up, and second that there was a difference with antihydrogen)," Fajans told Live Science's sister site Space.com in an email.
However, though the latest findings rule out theories that posit antimatter is repelled by gravity, only more precise measurements will tell if there is any difference in the gravitational force on antimatter compared to matter.
Nonetheless, by achieving the first direct observation of gravitational effects on antihydrogen, researchers mark the beginning of detailed and direct pursuit of the gravitational nature of antimatter, which remains puzzlingly scarce in the universe.
If matter and antimatter act so similarly, where's the universe's missing antimatter?
That is still an open question.
During the Big Bang, the universe is believed to have been rich with pairs of matter and antimatter particles, with the latter considered matter's mirror as its particles sport the same mass except for an opposite electrical charge. If matter and antimatter particles come into contact, they wipe out each other in a violent flash that leaves behind pure energy, so matter and antimatter particles are always created and destroyed in pairs.
In theory, that means the universe should feature nothing but leftover energy, at least according to the Standard Model of particle physics that outlines our current best understanding of how fundamental particles behave under the four fundamental forces of nature. But, that symmetry was broken down sometime during the evolution of the universe such that we clearly see matter dominating the observable universe. This is simply beyond what the Standard Model can explain. Thus, the processes that tipped the scales such that so little antimatter was left behind remain yet unknown.
"Unfortunately since our answers are consistent with general relativity, they do not shine any light on the scarcity of antimatter," Fajans told Space.com in an email. Fajans added that he anticipates the precision of the current experiment can be improved by a factor of 100 in the future. "This may lead to something new but of course we have no idea yet if that is to be the case. Most would say it is unlikely, but still worth pursuing."
The findings were published by the Antihydrogen Laser Physics Apparatus (ALPHA) collaboration at CERN on Wednesday (Sept. 27) in the journal Nature.
Originally posted on Space.com.
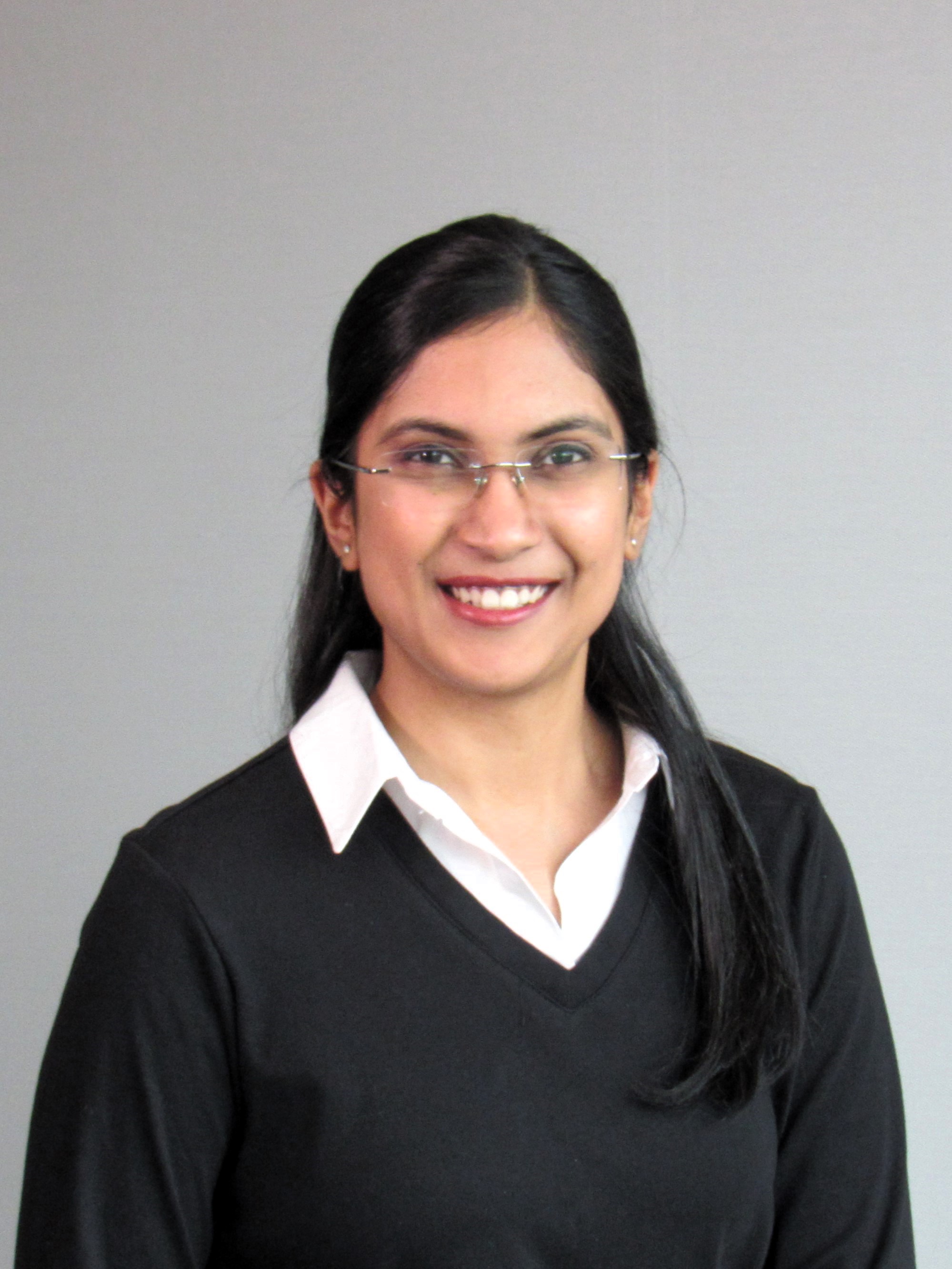
Sharmila Kuthunur is a Seattle-based science journalist focusing on astronomy and space exploration. Her work has also appeared in Scientific American, Astronomy and Space.com, among other publications. She has earned a master's degree in journalism from Northeastern University in Boston. Follow her on BlueSky @skuthunur.bsky.social