Einstein must be wrong: How general relativity fails to explain the universe
As new and powerful telescopes gather fresh data about the universe, they reveal the limits of older theories like Einstein's relativity.
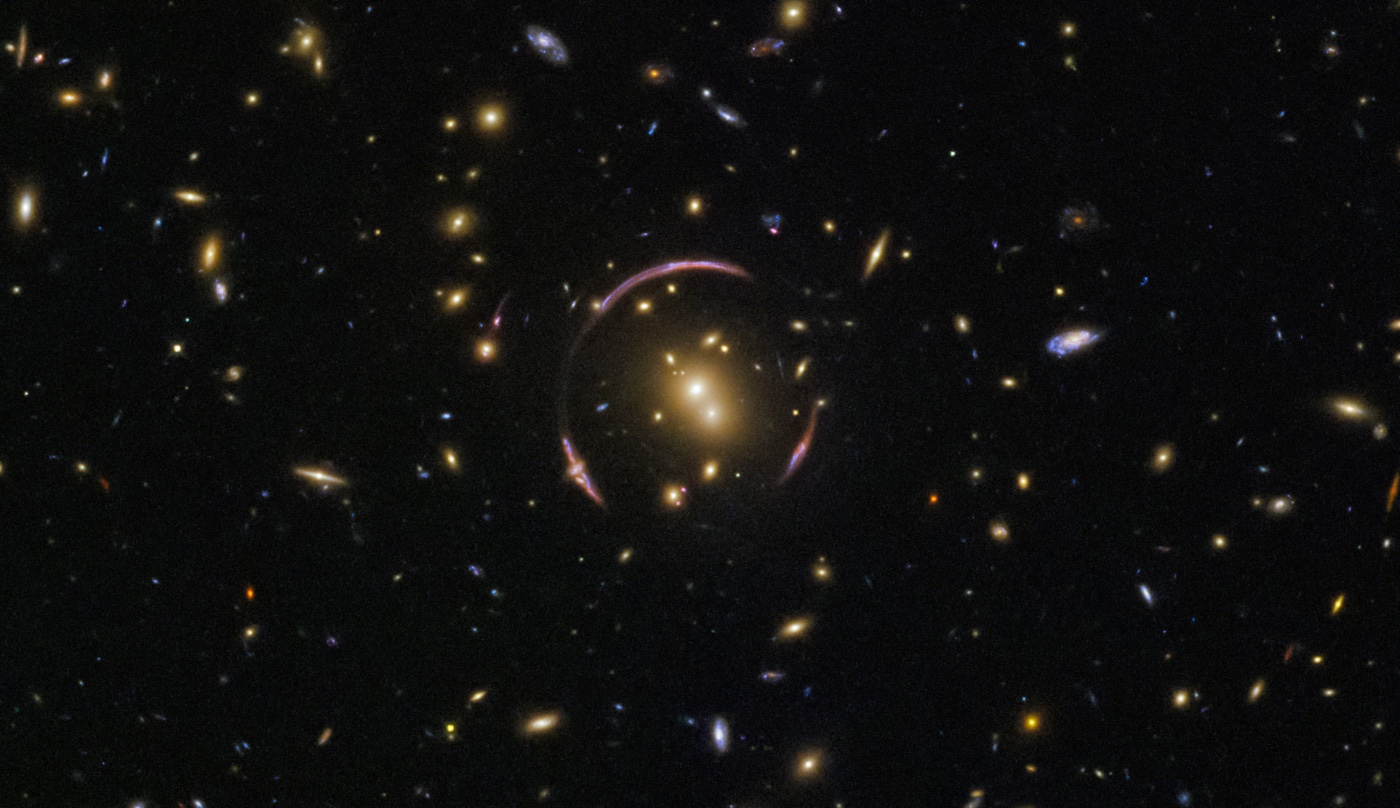
Einstein's theory of gravity — general relativity — has been very successful for more than a century. However, it has theoretical shortcomings. This is not surprising: the theory predicts its own failure at spacetime singularities inside black holes — and the Big Bang itself.
Unlike physical theories describing the other three fundamental forces in physics — the electromagnetic and the strong and weak nuclear interactions — the general theory of relativity has only been tested in weak gravity.
Deviations of gravity from general relativity are by no means excluded nor tested everywhere in the universe. And, according to theoretical physicists, deviation must happen.
Related: 10 discoveries that prove Einstein was right about the universe — and 1 that proves him wrong
Deviations and quantum mechanics
According to Einstein, our universe originated in a Big Bang. Other singularities hide inside black holes: Space and time cease to have meaning there, while quantities such as energy density and pressure become infinite. These signal that Einstein's theory is failing there and must be replaced with a more fundamental one.
Naively, spacetime singularities should be resolved by quantum mechanics, which apply at very small scales.
Quantum physics relies on two simple ideas: point particles make no sense; and the Heisenberg uncertainty principle, which states that one can never know the value of certain pairs of quantities with absolute precision — for example, the position and velocity of a particle. This is because particles should not be thought of as points but as waves; at small scales they behave as waves of matter.
Sign up for the Live Science daily newsletter now
Get the world’s most fascinating discoveries delivered straight to your inbox.
This is enough to understand that a theory that embraces both general relativity and quantum physics should be free of such pathologies. However, all attempts to blend general relativity and quantum physics necessarily introduce deviations from Einstein's theory.
Therefore, Einstein's gravity cannot be the ultimate theory of gravity. Indeed, it was not long after the introduction of general relativity by Einstein in 1915 that Arthur Eddington, best known for verifying this theory in the 1919 solar eclipse, started searching for alternatives just to see how things could be different.
Einstein's theory has survived all tests to date, accurately predicting various results from the precession of Mercury's orbit to the existence of gravitational waves. So, where are these deviations from general relativity hiding?
A century of research has given us the standard model of cosmology known as the Λ-Cold Dark Matter (ΛCDM) model. Here, Λ stands for either Einstein’s famous cosmological constant or a mysterious dark energy with similar properties.
Dark energy was introduced ad hoc by astronomers to explain the acceleration of the cosmic expansion. Despite fitting cosmological data extremely well until recently, the ΛCDM model is spectacularly incomplete and unsatisfactory from the theoretical point of view.
In the past five years, it has also faced severe observational tensions. The Hubble constant, which determines the age and the distance scale in the universe, can be measured in the early universe using the cosmic microwave background and in the late universe using supernovae as standard candles.
These two measurements give incompatible results. Even more important, the nature of the main ingredients of the ΛCDM model — dark energy, dark matter and the field driving early universe inflation (a very brief period of extremely fast expansion originating the seeds for galaxies and galaxy clusters) — remains a mystery.
From the observational point of view, the most compelling motivation for modified gravity is the acceleration of the universe discovered in 1998 with Type Ia supernovae, whose luminosity is dimmed by this acceleration. The ΛCDM model based on general relativity postulates an extremely exotic dark energy with negative pressure permeating the universe.
Problem is, this dark energy has no physical justification. Its nature is completely unknown, although a plethora of models has been proposed. The proposed alternative to dark energy is a cosmological constant Λ which, according to quantum-mechanical back-of-the-envelope (but questionable) calculations, should be huge.
However, Λ must instead be incredibly fine-tuned to a tiny value to fit the cosmological observations. If dark energy exists, our ignorance of its nature is deeply troubling.
Alternatives to Einstein's theory
Could it be that troubles arise, instead, from wrongly trying to fit the cosmological observations into general relativity, like fitting a person into a pair of trousers that are too small? That we are observing the first deviations from general relativity while the mysterious dark energy simply does not exist?
This idea, first proposed by researchers at the University of Naples, has gained tremendous popularity while the contending dark energy camp remains vigorous.
How can we tell? Deviations from Einstein gravity are constrained by solar system experiments, the recent observations of gravitational waves and the near-horizon images of black holes.
There is now a large literature on theories of gravity alternative to general relativity, going back to Eddington's 1923 early investigations. A very popular class of alternatives is the so-called scalar-tensor gravity. It is conceptually very simple since it only introduces one additional ingredient (a scalar field corresponding to the simplest, spinless, particle) to Einstein's geometric description of gravity.
The consequences of this program, however, are far from trivial. A striking phenomenon is the "chameleon effect," consisting of the fact that these theories can disguise themselves as general relativity in high-density environments (such as in stars or in the solar system) while deviating strongly from it in the low-density environment of cosmology.
As a result, the extra (gravitational) field is effectively absent in the first type of systems, disguising itself as a chameleon does, and is felt only at the largest (cosmological) scales.
The current situation
Nowadays the spectrum of alternatives to Einstein gravity has widened dramatically. Even adding a single massive scalar excitation (namely, a spin-zero particle) to Einstein gravity — and keeping the resulting equations "simple" to avoid some known fatal instabilities — has resulted in the much wider class of Horndeski theories, and subsequent generalizations.
Theorists have spent the last decade extracting physical consequences from these theories. The recent detections of gravitational waves have provided a way to constrain the physical class of modifications of Einstein gravity allowed.
However, much work still needs to be done, with the hope that future advances in multi-messenger astronomy lead to discovering modifications of general relativity where gravity is extremely strong.
This edited article is republished from The Conversation under a Creative Commons license. Read the original article.
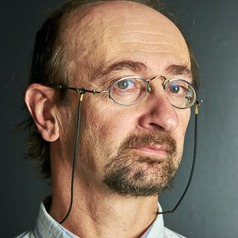
PhD in Astrophysics, supervisor George F.R. Ellis, worked in relativity, cosmology, and alternative theories of gravity for 30 years, been at Bishop's University for 16 years, currently full professor in the Physics & Astronomy Department. Author of 210 refereed journal articles and 7 books, funded by NSERC and volunteered extensively for NSERC, the Canadian Association of Physicists, and occasionally for other organizations worldwide.