Physicists harness atomic 'dark states' to store light
The new technique could be used to build networks from entangled atom clouds.
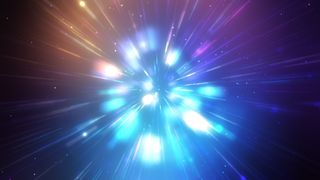
It turns out that what goes up needn't come back down.
Physicists have achieved a phenomenon known as subradiance, in which atoms linger in an excited state, in a dense cloud of atoms for the first time.
Harnessing subradiance could allow scientists to create reliable, long-lived quantum networks from clouds of atoms, the physicists reported in a new study.
Atoms gain energy by absorbing photons (light particles) that cause their electrons to jump from the lowest-energy "ground" state to higher-energy excited states. Once they're in an excited state, atoms spontaneously emit a photon and fall back to the ground state. But this isn't always the case. If many atoms are packed together and separated by a shorter distance than the wavelength of the emitted photon, the light they emit will cancel itself out, and the atoms will stay in their excited state.
Related: The 18 biggest unsolved mysteries in physics
This process, called subradiance, effectively staves off the decay of a large group, or "ensemble," of excited atoms. Subradiance has been observed before in dilute atomic ensembles and ordered atomic arrays, but never before in dense atomic clouds.
Subradiance works because of a phenomenon called destructive interference. When two waves of light with the same amplitude are made to occupy the same part of space, the peaks and the troughs of the waves can align to add together constructively, making a combined wave that is twice as bright, or destructively, cancelling out both waves entirely.
Sign up for the Live Science daily newsletter now
Get the world’s most fascinating discoveries delivered straight to your inbox.
But how can the canceling out of the light a cloud of atoms emits keep those atoms excited state? The key to understanding this idea, according to the researchers, is to observe subradiance quantum mechanics — the weird, probabilistic rules that govern the subatomic realm.
On the tiny scale of the weird quantum world, particles both have wave-like properties and can simultaneously travel all the infinite paths between one point and another. The path the particle "chooses" to take, and the one we observe, depends on how the wave-like particles interfere with themselves. It's not really the destructive interference between any emitted photons that traps atoms in excited states, but instead — and here's the wacky part — the possibility that it might happen, which stops the photons from being emitted in the first place.
"To understand what the probability of a physical event is, you need to sum all the paths leading to that event," co-author Loïc Henriet, a quantum software engineer at the French quantum processor company Pasqal, told Live Science in an email. "In some cases, paths interfere constructively and enhance the phenomenon, while in other cases, there are destructive interference effects that suppress the probability. The destructive interference of the photons that would have been emitted by individual atoms prevents the decay of an excited state collectively shared in the atomic ensemble."
To induce subradiance in a dense gas for the first time, the team confined a disordered cloud of cold rubidium atoms inside an optical tweezer trap. This technique, for which scientists won the Nobel prize in Physics in 2018, uses a highly concentrated beam of laser light to hold tiny particles in place. A second burst of laser light then excited the rubidium atoms.
Many of the excited atoms rapidly decayed through a process called superradiance, which is related to subradiance but instead has atoms combining their emitted light constructively into a super intense flash . But some atoms lingered in a subradiant, or "dark," state, unable to emit light that would destructively interfere. As time passed, some atoms in superradiant states also became subradiant, turning the atom cloud increasingly subradiant.
"We simply waited for the system to decay into dark states by itself," Henriet said. "The decay dynamic is rather complex, but we know that interactions somehow lead the system to populate subradiant states at a longer time."
Once they had found a way of making a subradiant cloud, the researchers jolted the atoms from their dark states by adjusting the optical tweezers, enabling the atoms to emit light without destructive interference. This resulted in a burst of light from the cloud.
The team also made multiple clouds of various shapes and sizes in order to study their properties. Only the number of atoms in an excited cloud affected its lifetime — the more atoms there were, the longer it took them to decay back to their ground states.
"Interference effects are collective effects; for it to happen, you need several emitters," Henriet said. "And it gets more pronounced when you increase the number of emitters. With only two atoms, it would be possible to have some sort of subradiance, but it would be a very small physical effect. By increasing the number of atoms, one can suppress photon emission more effectively."
Now that the researchers can make and control subradiant atom clouds, they plan to study techniques, such as arranging their clouds into regular geometric patterns, that, by enabling them to precisely tune the amount of interference they want, will give them even more control over the lifetimes of the excited atoms.
The researchers think that their discovery will aid in the development of many new technologies, such as new quantum computers and more precise weather prediction sensors.
The researchers published their findings May 10 in the journal Physical Review X.
Originally published on Live Science.
Ben Turner is a U.K. based staff writer at Live Science. He covers physics and astronomy, among other topics like tech and climate change. He graduated from University College London with a degree in particle physics before training as a journalist. When he's not writing, Ben enjoys reading literature, playing the guitar and embarrassing himself with chess.