Is dark matter made of 'Fermi balls' forged in the Big Bang?
The mysterious matter may have come from quantum bags that got squished together in the early universe.
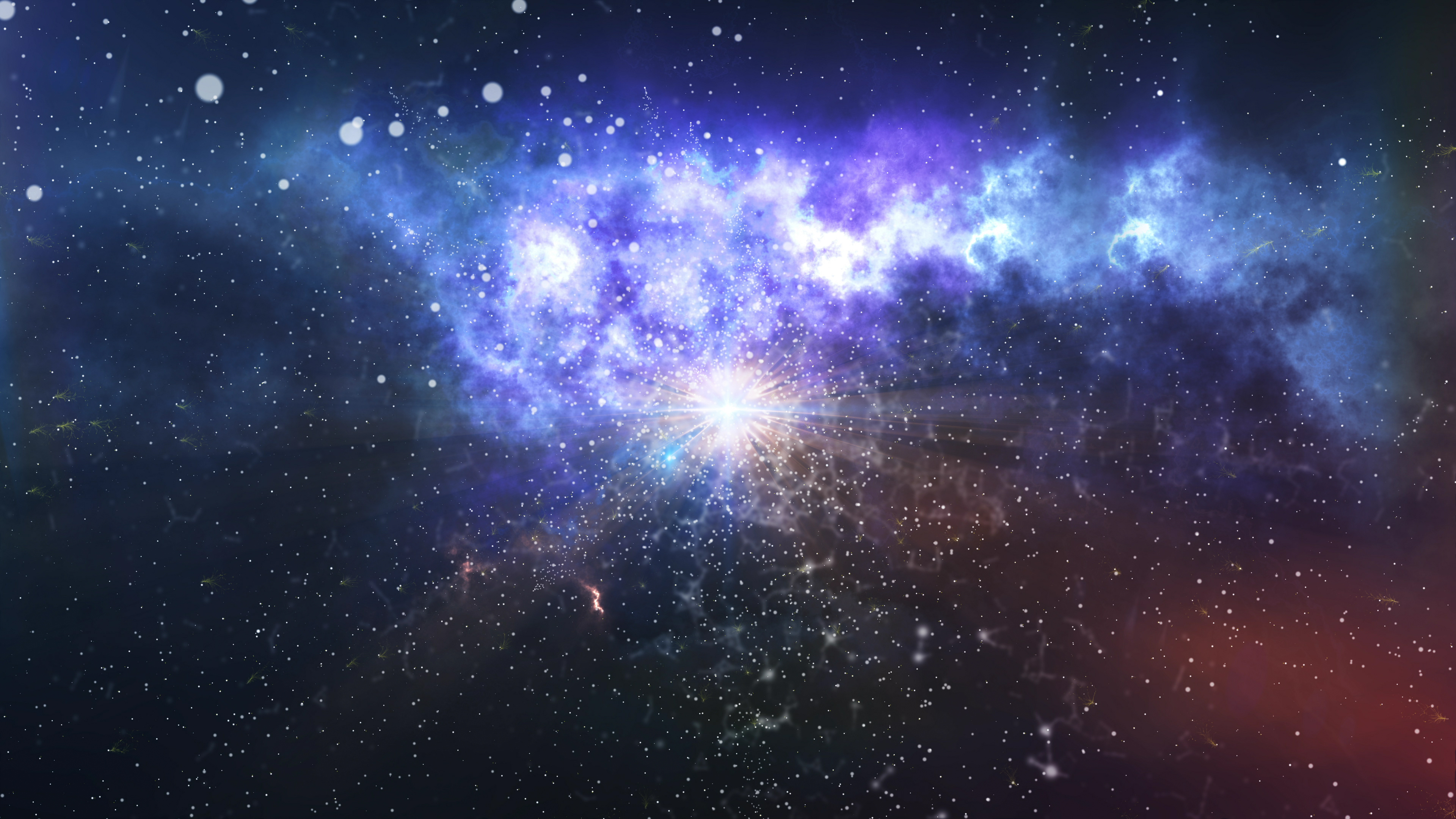
Dark matter — the mysterious substance that exerts gravity but doesn't interact with light — might be made of tiny black holes permeating the universe. And according to a new theory, those black holes might have been made from Fermi balls, or quantum "bags" of subatomic particles known as fermions that got smooshed together in dense pockets during the universe's infancy.
The theory could explain why dark matter came to dominate the universe.
"We find that in some cases, the Fermi balls are so dense that the fermions are too close to each other, triggering the collapse of a Fermi ball [in]to a black hole," Ke-Pan Xie, a researcher at the Center for Theoretical Physics at Seoul National University in South Korea, told Live Science.
Related: 10 huge findings about black holes
Xie and collaborator Kiyoharu Kawana, also of the Center for Theoretical Physics, have devised a new scenario to explain how dark matter came to dominate the universe: In the midst of an incredible transformation when the cosmos was less than a second old, a new kind of particle got trapped, collapsing to such a small point that they transformed into black holes. Those black holes then flooded the universe, providing the heft required to explain dark matter.
The case for primordial black holes
Astronomers and physicists cannot explain dark matter, the mysterious substance that makes up more than 80% of the mass of every large structure, from galaxies to the cosmic web itself, in the universe.
One intriguing possibility is that dark matter originated from black holes. After all, black holes, like dark matter, emit no light. "As a kind of nonluminous and compact object, black holes are a natural explanation for the dark matter," Xie said.
Sign up for the Live Science daily newsletter now
Get the world’s most fascinating discoveries delivered straight to your inbox.
But astronomers have known for a long time that normal, stellar-mass black holes can't explain the universe's dark matter. That's because not nearly enough stars have formed in the history of the universe to create enough black holes to account for the known dark matter.
But the earliest moments of the universe featured some pretty mind-boggling physics. Perhaps whatever was going on back then spawned trillions of smaller black holes. Those black holes could persist to the present day, potentially solving the dark matter riddle.
But to explain dark matter, the theory would have to make enough black holes.
A frothy universe
Xie and Kawana added several ingredients to their model, which is described in a paper published in June to the preprint database arXiv. (The paper has not yet been peer-reviewed.) They started with a very young, very hot, very dense universe. These extreme conditions allow some physical processes that do not happen in the normal conditions of the present-day universe.
The first ingredient is something called a scalar field, which is a quantum mechanical entity that encompasses all of space. (The well-known Higgs field, which gives matter its mass, is an example of one.) As the universe expanded and cooled, that scalar field underwent a phase transition, transforming from one quantum mechanical state to another.
That phase transition didn't happen all at once throughout the entire universe. Instead, there were a few points where the transition began from and then spread — just as a few bubbles in a pot of boiling water merge to form bigger bubbles, Xie said.
"This process is called a first-order phase transition: Water transfers from 'liquid phase' into 'gas phase,' and the latter first exists as growing bubbles," Xie said.
The new scalar field state, called the "ground state," spreads out from these points like a bunch of fizzing bubbles. Eventually, the bubbles merge completely, and the scalar field finishes its transition.
How to make a Fermi ball
To make primordial black holes that seed dark matter, however, Xie and Kawana needed another ingredient. So they added a new kind of fermion to their model. Fermions are a category of particles that make up the building blocks of the universe. For instance, the electrons, protons and neutrons that make up the atoms in your body are all fermions.
In the very early universe, these fermions moved freely within the scalar field. But according to the recipe that Xie and Kawana have cooked up, these fermions couldn't penetrate the little foaming bubbles of the new ground state of the cosmos as the phase transition proceeded.
As the bubbles grew, the fermions crowded into the remaining pockets, becoming Fermi balls. And that's when things went really haywire for them.
That's because there was an additional force, known as a Yukawa interaction, between the fermions, caused by that very same scalar field, Xie and Kawana proposed in the paper. Normally, fermions don't like to be crammed into small volumes together, but the scalar field added an attractive force that could overwhelm that natural repulsion, they theorized.
As an example, protons and neutrons are made of even tinier particles, called quarks. Quarks are fermions and normally hate each other, but an extra force, the strong force, glues them together. That force can be modeled as a Yukawa interaction, similar to the early-universe physics at play in Xie and Kawana's model.
Once the Yukawa attraction took hold, it was game over for the little Fermi balls, according to Xie and Kawana's theory. Wedged into little pockets of a rapidly changing universe, the clumps of fermions catastrophically collapsed, forming huge numbers of black holes.
Those black holes then survived through the end of the phase transition, going on to flood the universe as dark matter.
At least, that's the idea. It's a radical suggestion, but when it comes to the physics of the early universe — and the mystery surrounding dark matter — we need some radical suggestions, along with a healthy dose of observations, to make progress.
Originally published on Live Science.
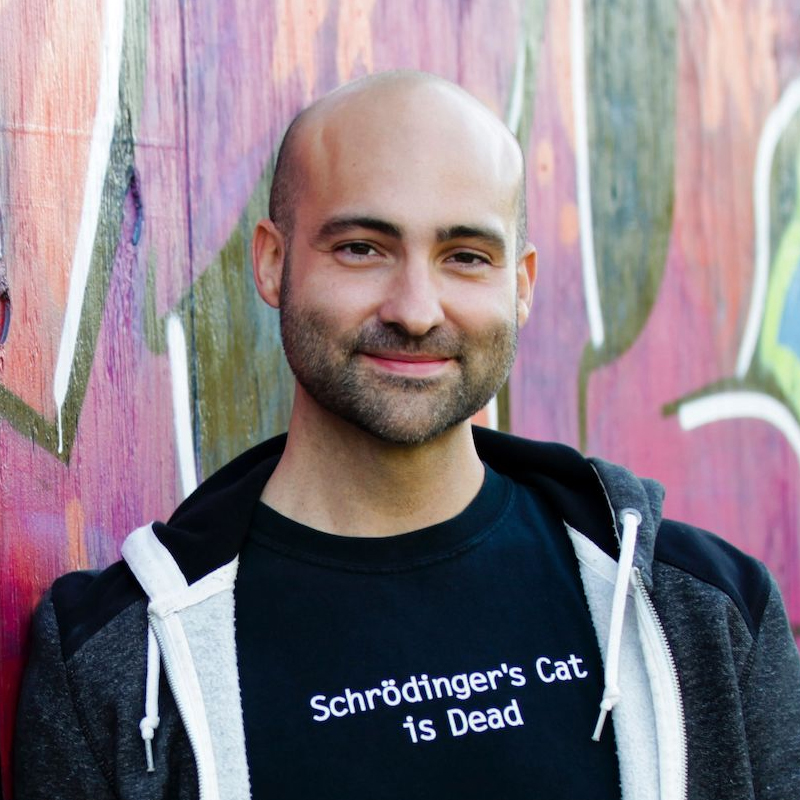
Paul M. Sutter is a research professor in astrophysics at SUNY Stony Brook University and the Flatiron Institute in New York City. He regularly appears on TV and podcasts, including "Ask a Spaceman." He is the author of two books, "Your Place in the Universe" and "How to Die in Space," and is a regular contributor to Space.com, Live Science, and more. Paul received his PhD in Physics from the University of Illinois at Urbana-Champaign in 2011, and spent three years at the Paris Institute of Astrophysics, followed by a research fellowship in Trieste, Italy.