Life Might Thrive 12 Miles Beneath Earth's Surface
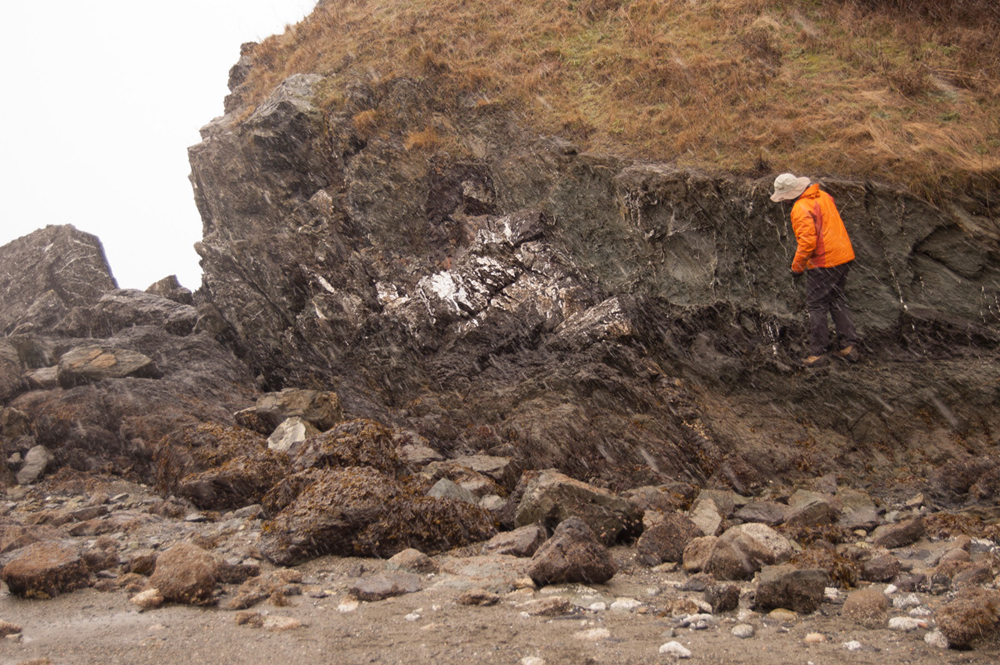
Life teems all over our planet's exterior and even down into the lightless oceanic depths. But just how far underground might life be able to hack it?
New research offers evidence of bacteria living as deep as 12 miles (19 kilometers) underground — quite possibly the deepest life has ever been glimpsed. Learning biology's terrestrial limits, though important in its own right, is critical to understanding life's rise on other planets with far less forgiving climates and surface conditionsthan the Earth's.
"Most studies report microbial life in the crust to no deeper than a few kilometers — just a mile or so," said Philippa Stoddard, an undergraduate in Yale University's geology and geophysics department. "Assuming our data are correct, this greatly expands our understanding of the extent of the Earth's biosphere." [Extreme Life on Earth: 8 Bizarre Creatures]
Stoddard presented the research at the Geological Society of America's annual meeting in Vancouver, British Columbia in October.
Acting on clues from nearly two-decades-old field work, Stoddard and her Yale colleagues examined rocks on Lopez Island in northwestern Washington. An outcrop there containing veins of the mineral aragonite, dredged up to the surface scores of millions of years ago by geological processes, was found to contain weirdly high levels of a lightweight version of the element carbon. This carbon signature is usually produced by microbes that excrete the carbon-containing compound methane.
The likeliest explanation is that lifeforms, once buried deep in the Earth's crust, altered the ancient aragonite's carbon signature. These microbes were so far underground they would have had to withstand extreme temperatures and pressures — a dramatic demonstration of life's robustness that bodes well for an ability to take hold in unearthly environments.
"I think that results like ours are very encouraging for the possibility of life on other planets," said Stoddard. "The more we learn about extreme environments on our own planet, the more we realize how resilient life is."
Get the world’s most fascinating discoveries delivered straight to your inbox.
Almost forgotten
The startling discovery initially cropped up in the 1990s. Fieldwork by J.G. Feehan for his 1997 doctoral dissertation with Yale professor Mark T. Brandon, who now is Stoddard's academic advisor, had identified the aragonite's very light carbon signatures.
Feehan suggested at the time that the signatures were the fingerprint of super-deep life. His focus, however, was on the geophysics of the rocks hosting the aragonite veins. So the subterranean life hypothesis sat, un-pursued, ever since.
Stoddard and Brandon, along with Yale professor Danny Rye, decided to pick up the thread. They recently returned to the scene in Washington State.
"Professor Brandon and I went back to the outcrop on Lopez Island where Feehan had done his isotopic measurements to see if we could corroborate his data and explore the suggestion of deep life more thoroughly," explained Stoddard. [The 7 Harshest Environments on Earth]
Telltale abundances
Specifically, as Feehan had done, Stoddard looked at the ratios of two carbon isotopes, or versions of an element containing different numbers of neutrons. The isotopes in question are carbon-12 and carbon-13, or C-12 and C-13. The former makes up the vast majority of carbon on the Earth. It has six protons and six neutrons in its atomic nucleus. C-13 has an extra, seventh neutron.
Life alters the typical ratio of C-12 to C-13 because most biochemical processes—eating, growth, and so on — divide isotopes into lighter and heavier camps. The way this works is actually pretty simple. Lower-numbered isotopes, possessing less mass, are lighter than higher-numbered isotopes. Lighter objects, like an empty cardboard box, are of course easier to move than a loaded safe of the same size. Similarly, lighter isotopes have an easier time getting about in the push-and-pull of biological matter at Lilliputian scales, driven by energy and molecular interactions.
"Because carbon-12 is the lighter isotope, it is more thermodynamically mobile than carbon-13," said Stoddard. "It can actually move faster."
Methane, a common waste product of microbes, contains a single carbon atom plus four hydrogen atoms. When microbes consume carbon-rich molecules and excrete methane, the waste methane containing the lighter, faster isotope C-12 returns to the environment more readily than C-13-laden methane. The typical ratio of one carbon isotope to the other ends up skewed as a result in rocks, for example, as in the case of the Lopez Island aragonite.
"The methane produced by microbes has much less of the heavy isotope than the standard ratio," said Stoddard.
Some non-biological processes can segregate carbon isotopes as well, but they tend not to do as efficiently, noted Stoddard.
The land down under
The San Juan Islands — including Lopez Island, site of the intriguing aragonite—only became islands as such about 100 million years ago, back in the dinosaurs' heyday. Before then, these sea-bottom rocks, located near what is now Vancouver Island, had subductedunder a neighboring chunk of rock, a geological process that often happens where tectonic plates meet at ocean and continental boundaries.
Buried in the bowels of the Earth, pressures and heat metamorphosed the dark basalt rock, creating thin, whitish veins of aragonite. Over time, microbes on the scene then slowly altered the carbon signatures in this aragonite through the methodical excretion of methane gas in this pitch-black, hot, squeezed environment.
Subsurface water trapped with the microbes could have further enabled their subsistence in such a place. The temperatures would likely have exceed 250 degrees Fahrenheit (121 degrees Celsius) — the known cutoff for even the hardiest of life to still function (in hot springs).
How would these microbes have survived? Counterintuitively, the exceedingly high pressure in a miles-deep habitat — in the neighborhood of 5,000 times the pressure exerted by Earth's atmosphere at sea level — could have helped. High pressures actually can stabilize biomolecules, such as DNA, offsetting the heat's destructive effects.
Similar scenarios could still persist today around the globe, meaning Earth's biosphere might extend many milesbelow the planet's surface.
"We've seen over the past couple decades of exploration that life can survive in an incredible diversity of ecosystems, even in deep-sea vents and glacial ice," said Stoddard. "If the deep earth was survivable for specialized microbes 100 million years ago, those same strategies could still work today." [Gallery: Creatures of Deep-Sea Vents]
Subsurface refuges
A similar approach could allow extraterrestrial life to get by under the desolate surfaces of worlds such as Mars.
Despite some of the obvious drawbacks of living deeply, microbes that have evolved to persist in such conditions would have advantages over life attempting to take hold aboveground in hostile environments.
Take Mars again as an example. Its surface gets bombarded with hundreds of times more cosmic radiationthan Earth's surface. Mars lacks a shielding magnetic field, so life developing on its surface would have substantially greater exposure to damaging radiation. Deep under the surface, that risk diminishes, along with other risks posed by, say, scalding or freezing temperatures.
"Underground environments would potentially be favorable locations for extraterrestrial life because they are more shielded from harmful surface conditions like cosmic radiation and insulated from extreme surface temperatures," said Stoddard. "It's definitely something we should keep in mind as we explore other planets."
Stoddard and colleagues intend to further study the long-buried Lopez Island rocks to glean more information about how, and if, life had indeed called them home.
"Although our isotope data is highly suggestive of deep life, there are still lots of things we don't know about this environment that could impact our conclusions," said Stoddard. "We're hoping to be able to construct a pretty detailed portrait of this deep ecosystem in the next couple of months."
This story was provided by Astrobiology Magazine, a web-based publication sponsored by the NASA astrobiology program.
Follow Space.com @Spacedotcom, Facebook and Google+.