Atoms' Quantum Spin Controlled in Odd Chilled Gas
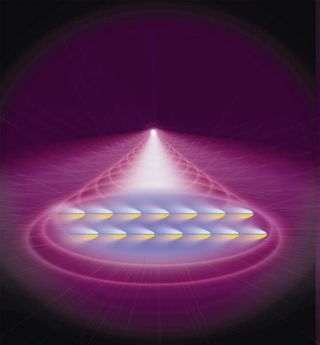
Physicists have revealed a new way to control the spins of atoms, an achievement that could open the way for new kinds of sensors while also shedding light on fundamental physics.
While scientists have been able to nudge the spins of atoms in the past, this new achievement, detailed in the June 6 issue of the journal Nature, is the first time they've done it in a strange chilled gas called a Bose-Einstein condensate.
The researchers say the finding may also be a step toward spintronics, or electronic circuits that use an electron's spin instead of its charge to carry information.
Chilled rubidium
The research team, from the Joint Quantum Institute, the National Institute for Standards and Technology (NIST) and the University of Maryland, used several lasers to trap rubidium atoms in a vacuum chamber. The rubidium atoms were in a tiny cloud, about 10 micrometers on a side, where 1 micrometer is about the size of a bacterium. The atoms were cooled to a few billionths of a degree above absolute zero. [Wacky Physics: The Coolest Little Particles in Nature]
By chilling the atoms, the researchers created a Bose-Einstein condensate, a special kind of gas in which all the atoms are in the same quantum mechanical state, meaning they all had either "up" or "down" spins; the condensate revealed phenomena that could ordinarily only be seen at the atomic scale.
In addition, very cold atoms are easier to track, since they are moving relatively slowly. At normal temperatures, the atoms move quickly and the apparatus has to be bigger. "You want to give yourself the time that ultracold atoms give you," said study researcher Ian Spielman, a NIST physicist. "And you can do the whole thing in less space."
Sign up for the Live Science daily newsletter now
Get the world’s most fascinating discoveries delivered straight to your inbox.
The researchers then used another set of lasers to gently push the cold gas. That small push moved the atoms just enough that the team could see the atoms with different spins, or magnetic alignments, move to one side or the other, depending on whether they were spinning up or down.
The movement is called the spin Hall effect. It involves particles of different spins moving to one side or the other of a piece of material when an electric current runs through it. The particles — they can be electrons or atoms — move perpendicular to the direction of the current.
Spin Hall effects have been detected before in semiconductors, but this is the first time an experiment has been done with a Bose-Einstein condensate.
By inducing this effect in the rubidium, the NIST team showed they could control where the atoms of different spins went, in this case by applying a laser.
Spintronics and sensors
The concept has other applications, one of which is "spintronics." A spintronic computer would be able to store more data and complete calculations more quickly than traditional computers.
While no one has come close to developing spintronic circuits, closer on the horizon are better inertial sensors, which detect acceleration and motion. Sophisticated ones are used in physics experiments, Spielman said. For instance, watching the atoms of different spins drift can show small variations in gravitational and magnetic fields.
As to fundamental physics, the experiment showed that since it's possible to induce the spin Hall effect, then it's also possible to induce its quantum-mechanical cousin. The quantum spin Hall effect describes a state of matter that exists in two-dimensional materials that are usually insulators rather than electrical conductors. It involves spin-up particles — usually electrons — conducting along one edge of the insulator, while spin-down electrons travel along the other side. That will be explored in a future set of experiments, Spielman said.
Matthew Beeler, lead author and now a staff scientist at the Johns Hopkins University Applied Physics Laboratory, said the experiment expands the "toolbox" available to scientists who want to manipulate particles and their spins. "The power is the ability to combine the tools in new ways. You could take spin Hall effect and add it to something else," Beeler said.
Follow us @livescience, Facebook & Google+. Original article on LiveScience.com.