New Device Points Way to Artificial Kidney Implants
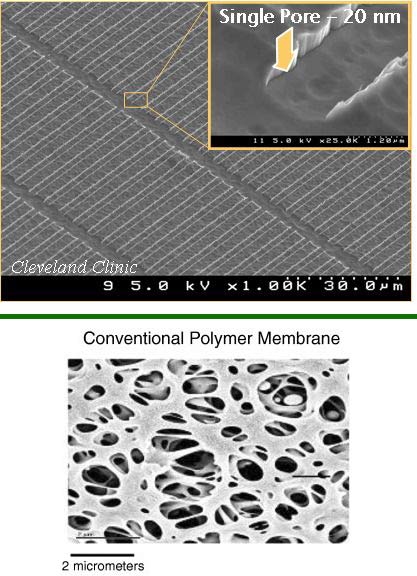
A new hi-tech membrane may soon improve the effectiveness of dialysis and might someday lead to implantable, artificial kidneys.
Though many obstacles remain, researchers say the breakthrough could revolutionize the approach to filtering blood in patients whose kidneys have failed.
The most common dialysis technique, hemodialysis, drains blood from the body, then filters and returns it. It is expensive, sometimes traumatic, and always time consuming. Most patients need several 3- to 4-hour sessions per week, most often in a medical facility.
A second, less frequently used technique, peritoneal dialysis (in which a special fluid is pumped into the patient's abdominal cavity so that the blood can be cleansed internally) shares many of the same problems, although it is less likely to require a medical facility for each session.
Just as troubling is that these kidney stand-ins aren't nearly as good as the real thing. They cleanse the blood of less than 20 percent of the waste that natural, healthy kidneys do.
Great need
Improving dialysis is essential, health experts say, because about 1.5 million people worldwide, including 325,000 in the United States, depend on dialysis to survive. And because people in most of the developed world are getting chubbier by the minute, diabetes—which is linked to obesity and is the cause of kidney failure in about 45 percent of cases— has earned "epidemic" status among many health scientists. According to some sources more than 18 million people in the United States alone have diabetes. The number of dialysis patients increases about 8 percent per year.
Sign up for the Live Science daily newsletter now
Get the world’s most fascinating discoveries delivered straight to your inbox.
Ideally, people for whom a kidney transplant isn't possible could receive an artificial organ that worked almost as well, said Dr. William Fissell, assistant professor of internal medicine at the University of Michigan School of Medicine.
3-step plan
The first step toward that goal, Fissell said, is improving the effectiveness of external artificial kidneys, or hemodialysis devices. Next would be to make an external device small enough for a patient to wear continuously. The final step would be a device that could be implanted, not unlike a pacemaker for the heart.
One of the keys to such a device, which Fissell and his colleagues, including Shuvo Roy, a biomedical engineer at the Cleveland Clinic's Lerner Research Institute are developing, is a much more effective filter.
"We think that we have a platform technology that will revolutionize the way that renal replacement is delivered," Fissell says.
Dialysis filters trap the good stuff (proteins and blood cells) and return it to the body while letting the bad stuff (toxins, excess fluids, and salt) through to be discarded.
The trick, Fissell says, is to refine the holes in the filter, which is a type of membrane. The holes need to be the right size, the right shape, and in the right pattern to let blood flow through the filter easily. They must be big enough to allow toxins to pass through the filter but not so big as to allow valuable proteins and blood cells to escape.
Under pressure
To trap the good stuff, current filters rely primarily on very small holes that are irregular in shape and are organized chaotically. Under a microscope they look like sponges.
But small holes means that blood must be forced through the filters with big, powerful pumps. And the chaotic patterns allow high-pressure-causing currents to form. These currents increase the pressure required to force blood through the filter.
A better membrane could be driven by a smaller, perhaps portable, pump. And an ideal membrane would work with normal blood pressure and so could be implanted into the body. The discarded toxins and other miscreants would be directed to bags attached to the patient.
Silicon approach
Fissell's team is building an easy-flow membrane by etching precise patterns into silicon wafers [image]. Micromachine technologies let the scientists increase the number of pores in a given area (to 10,000 pores per square millimeter) and control their shape (a slit) and pattern (undulating rows) to reduce turbulence in the blood. The next version of the membrane will have 10 times as many pores, about 100,000 per square millimeter, further reducing the amount of pressure required to force blood through it.
"Right now the real challenge is determining the correct pore size for the membranes and optimizing the biocompatibility of the membranes," Fissell explained. To perfect the membrane will require a better understanding of how natural kidneys filter the blood. Because the materials that a kidney's membranes —whether in a natural or artificial organ—must trap or let pass are biological, their interactions can be complex.
"It's not necessarily as simple as peas in a colander where the big peas stay and the little peas go," Fissell said.
And building a better membrane would just one of "tons" of barriers that must be bridged on the path to a practical implantable kidney, he cautions. Other factors that must be dealth with: biocompatibility, preventing the membranes from being fouled, finding the perfect pore size, and developing reliable manufacturing methods.
- Top 10 Mysterious Diseases
- The Appendix: Slimy But Not Worthless
- Men Donating Kidneys in Four-Way Swap
- Body Quiz: What the Parts Do
- Body Quiz: How the Parts Fit
- Body Quiz: The Parts List