How the First Life on Earth Struggled to Survive
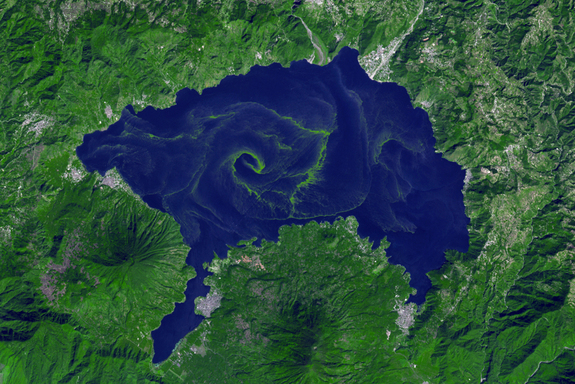
The earliest cells were unstable chemical systems that survived by combining a handful of shaky carbon-based assemblies together, researchers say.
All life on Earth is based on carbon. To create living matter from carbon, organisms carry out chemical reactions such as photosynthesis to generate organic compounds from the carbon dioxide in the environment. These mechanisms, known as carbon fixation, make up the largest bridge between Earth's nonliving chemistry and its life.
All organisms that fix carbon do so in one of six ways. It was unclear as to which of the six types came first, and how their development was linked with environmental and biological changes. Mapping the evolution of this vital chemistry would shed light on the roots of life on Earth.
Now scientists have traced the six methods of carbon fixation seen in modern life back to what may have been a single ancestral form.
Researchers created family trees for carbon-fixing mechanisms by looking at the collections of genes and aspects of metabolism underlying them and seeing how similar or different they were. From these patterns of relatedness, they reconstructed the complete evolutionary history of carbon fixation.
The earliest life that carried out carbon fixation apparently combined multiple carbon-fixing mechanisms together. This redundancy, not seen in modern cells, gave early life a robustness that compensated for the lack of refined control it had over its internal chemistry. [Photos: 8 Extreme Creatures]
"It seems likely that the earliest cells were rickety assemblies whose parts were constantly malfunctioning and breaking down," said researcher Eric Smith, a physicist at the Santa Fe Institute. "How can any metabolism be sustained with such shaky support? The key is concurrent and constant redundancy."
Get the world’s most fascinating discoveries delivered straight to your inbox.
This redundancy was the foundation of later splits that created the earliest major branches in the tree of life. For example, the first major life-form split — between the branch holding the ancestors of blue–green algae and most other bacteria and the branch that includes Archaea, the other major early group of single-celled microorganisms — came with the earliest appearance of oxygen on Earth. Back then oxygen was toxic to organisms, since they had no way to make use of it. The peril oxygen posed caused these branches to diverge — these lineages apparently confronted oxygen at different points in history, after they had pursued different evolutionary paths, leading to different ways of responding to oxygen.
Once early cells had more refined chemistries — for instance, with membranes that could better control their contents, or enzymes that were better at controlling specific reactions — life developed in predictable ways in response to environmental factors such as increased alkalinity from carbonates. This stands in contrast to the common belief that luck dominated evolutionary innovations, and that rewinding and replaying evolutionary history might lead to a radically different tree of life.
"It appears basic chemistry and physics play an enormous role in the structure of early evolution, as opposed to things like random chance," researcher Rogier Braakman, a chemist at the Santa Fe Institute, told LiveScience.
It remains uncertain whether there was a single ancestral organism that possessed all these carbon-fixation mechanisms, Braakman said. "It could be that there was a community of tightly coupled organisms that regularly swapped their parts, which specific lineages came from later," he said. "Our approach does not distinguish between a single organism or a community of organisms at this point."
The researchers plan to look at other metabolic pathways, amino acid synthesis, to shed light on the larger picture of early evolution.
The scientists detailed their findings this week in the journal PLoS Computational Biology.
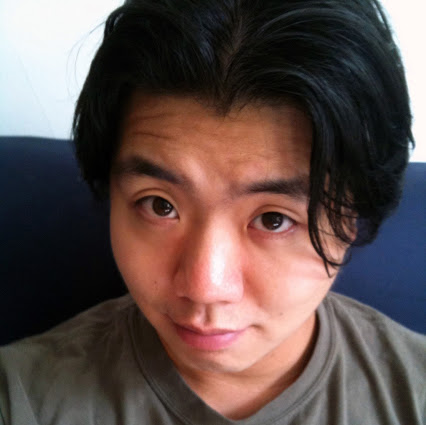