Warp drive experiment to turn atoms invisible could finally test Stephen Hawking's most famous prediction
The Unruh effect has been predicted half a century
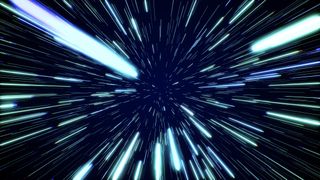
A new warp speed experiment could finally offer an indirect test of famed physicist Stephen Hawking's most famous prediction about black holes.
The new proposal suggests that, by nudging an atom to become invisible, scientists could catch a glimpse of the ethereal quantum glow that envelops objects traveling at close to the speed of light.
The glow effect, called the Unruh (or Fulling-Davies-Unruh) effect, causes the space around rapidly accelerating objects to seemingly be filled by a swarm of virtual particles, bathing those objects in a warm glow. As the effect is closely related to the Hawking effect — in which virtual particles known as Hawking radiation spontaneously pop up at the edges of black holes — scientists have long been eager to spot one as a hint of the other’s existence.
Related: 'X particle' from the dawn of time detected inside the Large Hadron Collider
But spotting either effect is incredibly hard. Hawking radiation only occurs around the terrifying precipice of a black hole, and achieving the acceleration needed for the Unruh effect would probably need a warp drive. Now, a groundbreaking new proposal, published in an April 26 study in the journal Physical Review Letters, could change that. Its authors say they have uncovered a mechanism to dramatically boost the strength of the Unruh effect through a technique that can effectively turn matter invisible.
"Now at least we know there is a chance in our lifetimes where we might actually see this effect," co-author Vivishek Sudhir, an assistant professor of mechanical engineering at MIT and a designer of the new experiment, said in a statement. "It’s a hard experiment, and there’s no guarantee that we’d be able to do it, but this idea is our nearest hope."
First proposed by scientists in the 1970s, the Unruh effect is one of many predictions to come out of quantum field theory. According to this theory, there is no such thing as an empty vacuum. In fact, any pocket of space is crammed with endless quantum-scale vibrations that, if given sufficient energy, can spontaneously erupt into particle-antiparticle pairs that almost immediately annihilate each other. And any particle — be it matter or light — is simply a localized excitation of this quantum field.
Sign up for the Live Science daily newsletter now
Get the world’s most fascinating discoveries delivered straight to your inbox.
In 1974, Stephen Hawking predicted that the extreme gravitational force felt at the edges of black holes — their event horizons — would also create virtual particles.
Gravity, according to Einstein’s theory of general relativity, distorts space-time, so that quantum fields get more warped the closer they get to the immense gravitational tug of a black hole’s singularity. Because of the uncertainty and weirdness of quantum mechanics, this warps the quantum field, creating uneven pockets of differently moving time and subsequent spikes of energy across the field. It is these energy mismatches that make virtual particles emerge from what appears to be nothing at the fringes of black holes.
"Black holes are believed to be not entirely black," lead author Barbara Šoda, a doctoral student in physics at the University of Waterloo in Canada, said in a statement. "Instead, as Stephen Hawking discovered, black holes should emit radiation."
Much like the Hawking effect, the Unruh effect also creates virtual particles through the weird melding of quantum mechanics and the relativistic effects predicted by Einstein. But this time, instead of the distortions being caused by black holes and the theory of general relativity, they come from near light-speeds and special relativity, which dictates that time runs slower the closer an object gets to the speed of light.
According to quantum theory, a stationary atom can only increase its energy by waiting for a real photon to excite one of its electrons. To an accelerating atom, however, fluctuations in the quantum field can add up to look like real photons. From an accelerating atom’s perspective, it will be moving through a crowd of warm light particles, all of which heat it up. This heat would be a telltale sign of the Unruh effect.
But the accelerations required to produce the effect are far beyond the power of any existing particle accelerator. An atom would need to accelerate to the speed of light in less than a millionth of a second — experiencing a g force of a quadrillion meters per second squared — to produce a glow hot enough for current detectors to spot.
"To see this effect in a short amount of time, you’d have to have some incredible acceleration," Sudhir said. "If you instead had some reasonable acceleration, you’d have to wait a ginormous amount of time — longer than the age of the universe — to see a measurable effect."
To make the effect realizable, the researchers proposed an ingenious alternative. Quantum fluctuations are made denser by photons, which means that an atom made to move through a vacuum while being hit by light from a high-intensity laser could, in theory, produce the Unruh effect, even at fairly small accelerations. The problem, however, is that the atom could also interact with the laser light, absorbing it to raise the atom's energy level, producing heat that would drown out the heat generated by the Unruh effect.
But the researchers found yet another workaround: a technique they call acceleration-induced transparency. If the atom is forced to follow a very specific path through a field of photons, the atom will not be able to "see" the photons of a certain frequency, making them essentially invisible to the atom. So by daisy-chaining all these workarounds, the team would then be able to test for the Unruh effect at this specific frequency of light.
Making that plan a reality will be a tough task. The scientists plan to build a lab-size particle accelerator that will accelerate an electron to light speeds while hitting it with a microwave beam. If they’re able to detect the effect, they plan to conduct experiments with it, especially those that will enable them to explore the possible connections between Einstein's theory of relativity and quantum mechanics.
"The theory of general relativity and the theory of quantum mechanics are currently still somewhat at odds, but there has to be a unifying theory that describes how things function in the universe," co-author Achim Kempf, a professor of applied mathematics at the University of Waterloo, said in a statement. "We've been looking for a way to unite these two big theories, and this work is helping to move us closer by opening up opportunities for testing new theories against experiments."
Originally published on Live Science.
Ben Turner is a U.K. based staff writer at Live Science. He covers physics and astronomy, among other topics like tech and climate change. He graduated from University College London with a degree in particle physics before training as a journalist. When he's not writing, Ben enjoys reading literature, playing the guitar and embarrassing himself with chess.