
'Bumblebee gravity' could explain why the universe is expanding so quickly
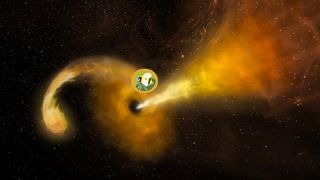
Physicists have long assumed that the universe is pretty much the same in any direction, and now they've found a new way to test that hypothesis: by examining the shadow of a black hole.
If that shadow is a wee bit smaller than existing physics theories predict, it could help prove a far-out notion called bumblebee gravity, which describes what would happen if the seemingly perfect symmetry of the universe isn't so perfect after all.
If scientists can find a black hole with such an undersized shadow, it would open the door to a brand-new understanding of gravity — and perhaps explain why the universe is expanding ever faster.
But to understand how this bumblebee idea could fly, let's dig into some fundamental physics.
Related: The 18 biggest unsolved mysteries in physics
Looking in the mirror
Physicists love symmetry; after all, it helps us understand some of the deepest secrets of the universe. For example, physicists have realized that if you conduct an experiment on fundamental physics you can move your testing equipment somewhere else and you'll get the same result again (that is, if all other factors, like the temperature and the strength of gravity, remain the same).
In other words, no matter where in space you conduct your experiment, you'll get the same result. Through mathematical logic, this leads directly to the law of conservation of momentum.
Sign up for the Live Science daily newsletter now
Get the world’s most fascinating discoveries delivered straight to your inbox.
Another example: If you run your experiment and wait awhile before running it again, you'll get the same result (again, all else being equal). This temporal symmetry leads directly to the law of conservation of energy — that energy can never be created nor destroyed.
There's another important symmetry that forms a bedrock of modern physics. It's called the "Lorentz" symmetry, in honor of Hendrik Lorentz, the physicist who figured all this out in the early 1900s. It turns out that you can take your experiment and turn it, and (all else being equal) you will get the same result. You can also boost your experiment to a fixed velocity and still get the same result.
In other words, all else being equal — and yes, I'm repeating that often, because it's important — if you're conducting an experiment at total rest, and doing the same experiment at half the speed of light, you'll get the same result.
This is the symmetry that Lorentz uncovered: The laws of physics are the same regardless of position, time, orientation and speed.
What do we get out of this fundamental symmetry? Well, for starters, we get Einstein's entire theory of special relativity, which sets out a constant speed of light and explains how space and time are linked for objects traveling at different speeds.
Bumblebee gravity
Special relativity is so essential to physics that it's almost a metatheory of physics: If you want to concoct your own idea of how the universe works, it has to be compatible with the dictates of special relativity.
Or not.
Physicists are constantly trying to cook up new and improved theories of physics, because the old ones, like general relativity, which describes how matter warps space-time and the Standard Model of particle physics, cannot explain everything in the universe, such as what happens at the heart of a black hole. And one very juicy place to look for new physics is to see if any cherished notions might not be so accurate in extreme conditions — cherished notions like Lorentz symmetry.
Related: 8 ways you can see Einstein's theory of relativity in real life
Some models of gravity argue that the universe isn't exactly symmetrical after all. These models predict that there are extra ingredients in the universe that force it to not exactly obey Lorentz symmetry all the time. In other words, there would be a special, or privileged, direction in the cosmos.
These new models describe a hypothesis dubbed "bumblebee gravity." It gets its name from the supposed idea that scientists once claimed that bumblebees shouldn't be able to fly, because we didn't understand how their wings generated lift. (Scientists never actually believed that, by the way.) We don't fully understand how these models of gravity work and how they could be compatible with the universe that we see, and yet, there they are, staring us in the face as viable options for new physics.
One of the most powerful uses of bumblebee gravity models is to potentially explain dark energy —the phenomenon responsible for the observed accelerated expansion of the universe. It turns out that the degree to which our universe violates Lorentz symmetry can be tied to an effect that generates accelerated expansion. And because we have no idea what's creating dark energy, this possibility looks very appealing indeed.
The black shadow
So you have a buzzy new theory of gravity based on some icon-smashing ideas like symmetry violation. Where would you go to test that idea? You'd go to the place where gravity is stretched to the absolute limit: a black hole. In the new study, not yet peer-reviewed and published online in November 2020 to the preprint database arXiv, researchers did just that, looking at the shadow of a black hole in a hypothetical universe modeled to be as realistic as possible.
(Remember that first-ever image of black hole M87, produced by the Event Horizon Telescope just a year ago? That hauntingly beautiful, dark void in the center of the bright ring was actually the black hole's "shadow," the region that sucked in all of the light from behind and around it.)
To make the model as realistic as possible, the team placed a black hole in the background of a universe that was accelerating in its expansion (exactly like what we observe) and tuned the level of symmetry violation to match the behavior of dark energy that scientists measure.
They found that, in this case, a black hole's shadow can appear up to 10% smaller than it would in a "normal-gravity" world, providing a clear way to test bumblebee gravity. While the current image of black hole M87 is too fuzzy to tell the difference, efforts are underway to take even better pictures of more black holes, probing some of the deepest mysteries of the universe in the process.
Originally published on Live Science.
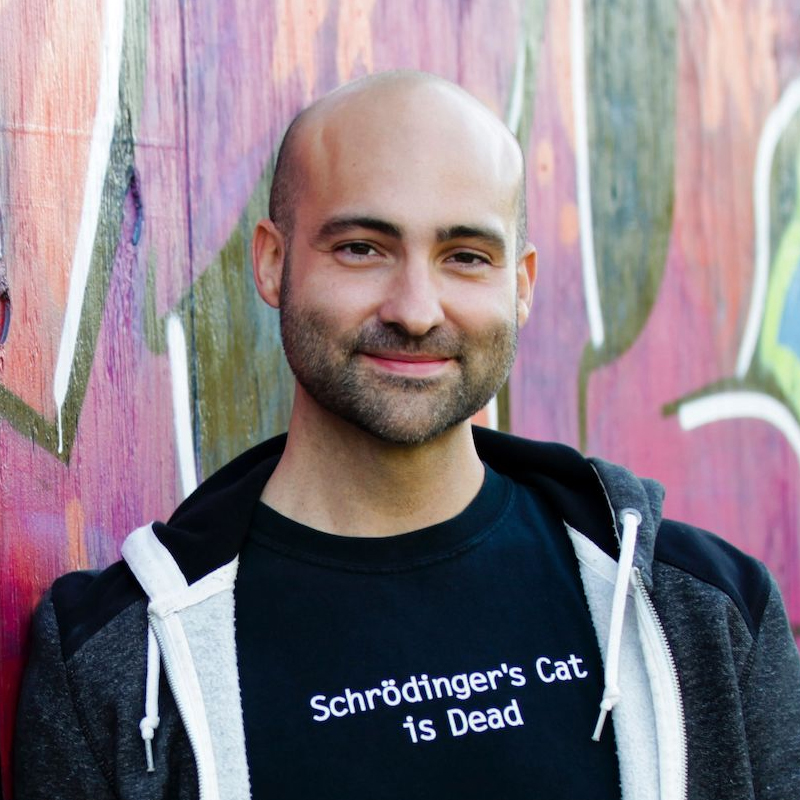
Paul M. Sutter is a research professor in astrophysics at SUNY Stony Brook University and the Flatiron Institute in New York City. He regularly appears on TV and podcasts, including "Ask a Spaceman." He is the author of two books, "Your Place in the Universe" and "How to Die in Space," and is a regular contributor to Space.com, Live Science, and more. Paul received his PhD in Physics from the University of Illinois at Urbana-Champaign in 2011, and spent three years at the Paris Institute of Astrophysics, followed by a research fellowship in Trieste, Italy.