Twisted light from the beginning of time could reveal brand-new physics
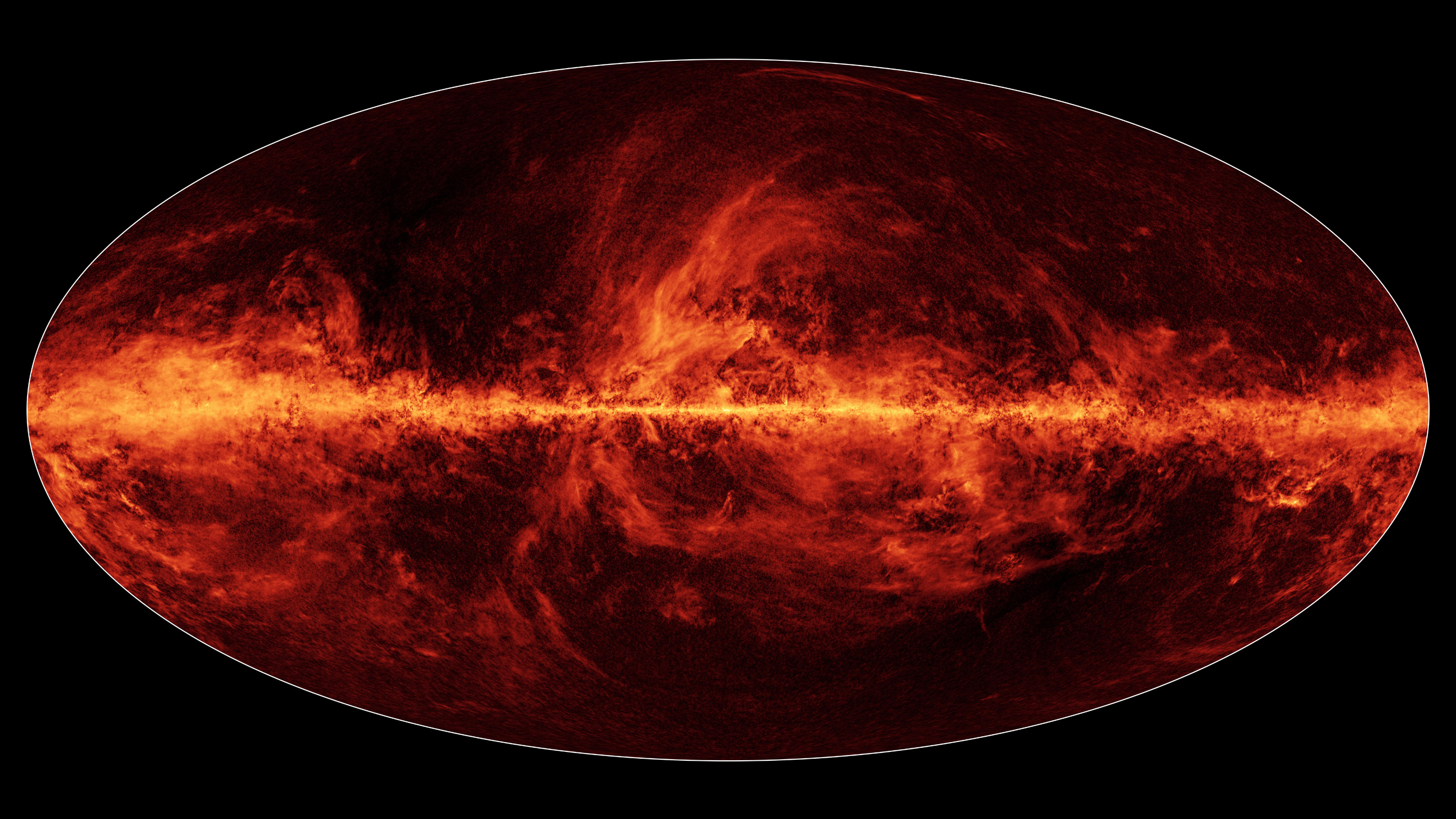
A twist in the universe's first light could hint that scientists need to rethink physics.
A pair of Japanese scientists looked at the polarization or orientation of light from the cosmic microwave background radiation, some of the earliest light emitted after the universe's birth. They found the polarization of photons, or light particles, might be slightly rotated from their original orientation when the light was first produced. And dark energy or dark matter may have been responsible for that rotation. (Dark energy is a hypothetical force that is flinging the universe apart, while proposed dark matter is a substance that exerts gravitational pull yet does not interact with light.)
The rotated signature of the photon polarization tells the scientists that something may have interacted with those photons — specifically something that violates a symmetry physicists call parity. This symmetry or parity says that everything looks and behaves the same way, even in a flipped system — similar to how things look in the mirror. And if the system was following this parity rule, there wouldn't be this rotation change.
Related: From Big Bang to present: snapshots of our universe through time
Parity is shown by all subatomic particles and all forces except the weak force. However, the new results suggest that whatever the early light might have interacted with might be violating this parity.
"Maybe there is some unknown particle, which contributes to dark energy, that perhaps rotates the photon polarization," said study lead author Yuto Minami, a physicist at the Institute of Particle and Nuclear Studies (IPNS) of High Energy Accelerator Research Organization (KEK) in Japan.
When the cosmic microwave background radiation, or CMB, was first emitted 13.8 billion years ago, it was polarized in the same direction. Looking at how the light's polarization has rotated over time allows scientists to probe the universe's history since then, by looking at how the light has changed as it travels across space and time.
Sign up for the Live Science daily newsletter now
Get the world’s most fascinating discoveries delivered straight to your inbox.
Previously, scientists have studied the CMB's polarization and how it's been rotated over time, but they weren't able to measure it accurately enough to study parity because of large uncertainty in the calibration of the detectors that measure the photon's polarization. In the new study, reported Nov. 23 in the journal Physical Review Letters, researchers figured out a way to precisely measure the rotation of the instruments by using another source of polarized light — dust from within the Milky Way. Because this light hasn't traveled as far, it's likely not strongly affected by dark energy or dark matter.
Using the dusty Milky Way light, the scientists were able to figure out precisely how their instruments were oriented, so they knew the rotation in the light was real, not something caused by their instruments. This allowed them to determine the polarization rotation of CMB light was non-zero, which means that the light has interacted with something that violates parity. It's possible something in the early universe affected the light, but it's more likely that it was something along the light's path as it traveled toward Earth, Minami told Live Science.
That something could be dark energy or dark matter, which would mean that the particles that make up these mysterious substances violate parity.
The authors reported their findings with 99.2% confidence, meaning there's an 8 in 1,000 chance of getting similar results by chance. However, this isn't quite as confident as physicists require for absolute proof. For that, they need five sigma, or 99.99995% confidence, which likely isn't possible with data from just one experiment. But future and existing experiments might be able to gather more accurate data, which could be calibrated with the new technique to reach a high-enough level of confidence.
"Our results do not mean a new discovery," Minami said. "Only that we found a hint of it."
Originally published on Live Science.
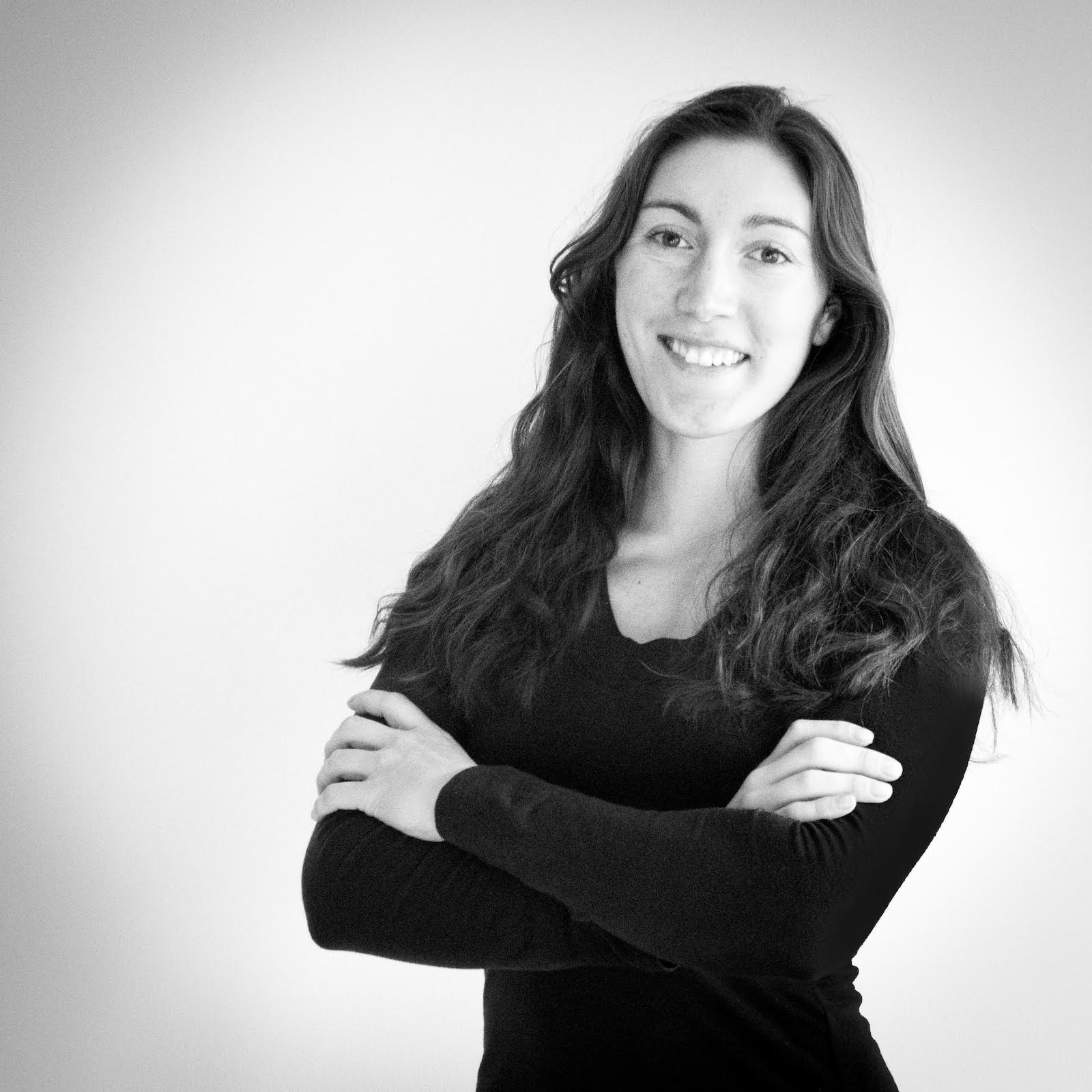
Mara Johnson-Groh is a contributing writer for Live Science. She writes about everything under the sun, and even things beyond it, for a variety of publications including Discover, Science News, Scientific American, Eos and more, and is also a science writer for NASA. Mara has a bachelor's degree in physics and Scandinavian studies from Gustavus Adolphus College in Minnesota and a master's degree in astronomy from the University of Victoria in Canada.