Did German physicists accidentally discover dark matter in 2014?
What if dark matter was sitting in data from a German laboratory all along?
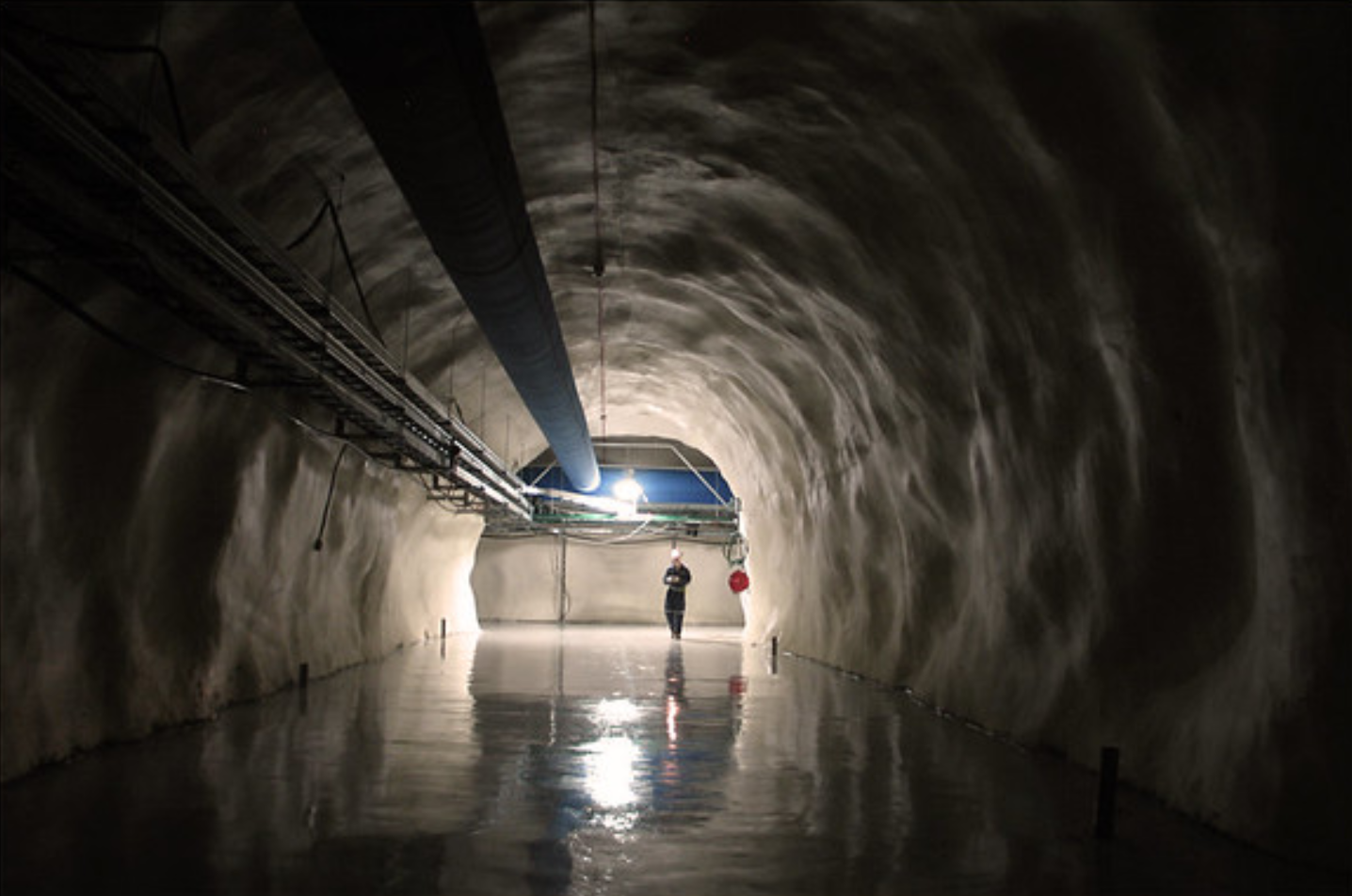
Could we have already discovered dark matter?
That's the question put forth in a new paper published Feb.12 in the Journal of Physics G. The authors outlined how dark matter might be made of a particle known as the d*(2380) hexaquark, which was likely detected in 2014.
Dark matter, which exerts gravitational pull but emits no light, isn't something anyone's ever touched or seen. We don't know what it's made of, and countless searches for the stuff have come up empty. But an overwhelming majority of physicists are convinced it exists. The evidence is plastered all over the universe: Clusters of stars spinning far faster than they otherwise should, mysterious distortions of light across the night sky, and even holes punched in our galaxy by an unseen impactor point to something being out there — making up most of the mass of the universe — that we don't yet understand.
Most widely studied theories of dark matter involve whole classes of never-before-seen particles from well outside the Standard Model of physics, the dominant theory describing subatomic particles. Most of these fit into one of two categories: the lightweight axions and the heavyweight WIMPs, or weakly interacting massive particles. There are other, more exotic theories involving as-yet undiscovered species of neutrinos or a theoretical class of microscopic black holes. But rarely does anyone propose that dark matter is made of something we already know exists.
Related: The 11 biggest unanswered questions about dark matter
Mikhail Bashkanov and Daniel Watts, physicists at the University of York in England, broke that mold, arguing that the d*(2380) hexaquark, or "d-star," could explain all the missing matter.
Quarks are fundamental physical particles in the Standard Model. Three of them bound together (using particles known as gluons) can make a proton or a neutron, the building blocks of atoms. Arrange them in other ways and you get different, more exotic particles. The d-star is a positively charged, six-quark particle that researchers believe existed for a sliver of a second during a 2014 experiment at Germany's Jülich Research Center. Because it was so fleeting, that d-star detection hasn't been absolutely confirmed.
Get the world’s most fascinating discoveries delivered straight to your inbox.
Individual d-stars couldn't explain dark matter because they don't last long enough before decaying. However, Bashkanov told Live Science, early in the universe's history, the particles might have clumped together in a way that would have kept them from decaying.
That scenario occurs with neutrons. Take a neutron out of a nucleus, and it very quickly decays, but mix it with other neutrons and protons inside the nucleus, and it becomes stable, Bashkanov said.
"Hexaquarks behave in exactly the same way," Bashkanov said.
Bashkanov and Watts theorized that groups of d-stars could form substances known as Bose-Einstein condensates, or BECs. In quantum experiments, BECs form when temperatures drop so low that atoms begin to overlap and blend together, a bit like the protons and neutrons inside atoms. It's a state of matter distinct from solid matter.
Early in the universe's history, those BECs would have captured free electrons, forming a neutrally charged material. A neutrally charged d-star BEC, the physicists wrote, would behave a lot like dark matter: invisible, slipping through luminous matter without noticeably bumping it around, yet exerting significant gravitational pull on the surrounding universe.
The reason you don't fall through a chair when you sit on it is that the electrons of the chair push against the electrons of your backside, creating a barrier of negative electric charges that refuse to cross paths. Under the right conditions, Bashkanov said, BECs made of hexaquarks with trapped electrons would have no such barriers, slipping through other kinds of matter like perfectly neutral ghosts.
These BECs might have formed soon after the Big Bang, as space transitioned from a sea of hot quark-gluon plasma with no distinct atomic particles into our modern era with particles like protons, neutrons and their cousins. At the moment when those basic atomic particles formed, conditions were perfect for hexaquark BECs to precipitate from the quark-gluon plasma.
"Before this transition, the temperature is too high; after it, the density is too low," Bashkanov said.
During this transition period, the quarks could have frozen into either ordinary particles, such as protons and neutrons, or into the hexaquark BECs that today might make up dark matter, Bashkanov said. If these hexaquarks BECs are out there, the researchers wrote, we might be able to detect them. Even though the BECs are quite long-lived, they will occasionally decay around Earth. And that decay would show up as a particular signature in detectors designed to spot cosmic rays, and appear as if it were coming from every direction at once as if the source filled all of space.
The next step, they wrote, is to look for that signature.
- The 18 biggest unsolved mysteries in physics
- The large numbers that define the universe
- Twisted physics: 7 mind-blowing findings
Originally published on Live Science.
OFFER: Save at least 53% with our latest magazine deal!
With impressive cutaway illustrations that show how things function, and mindblowing photography of the world’s most inspiring spectacles, How It Works represents the pinnacle of engaging, factual fun for a mainstream audience keen to keep up with the latest tech and the most impressive phenomena on the planet and beyond. Written and presented in a style that makes even the most complex subjects interesting and easy to understand, How It Works is enjoyed by readers of all ages.
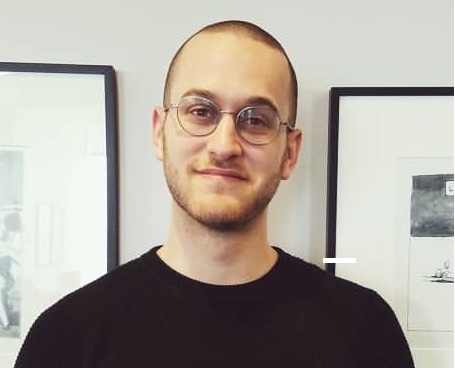