Does Your Brain Let You Hear Your Own Footsteps?
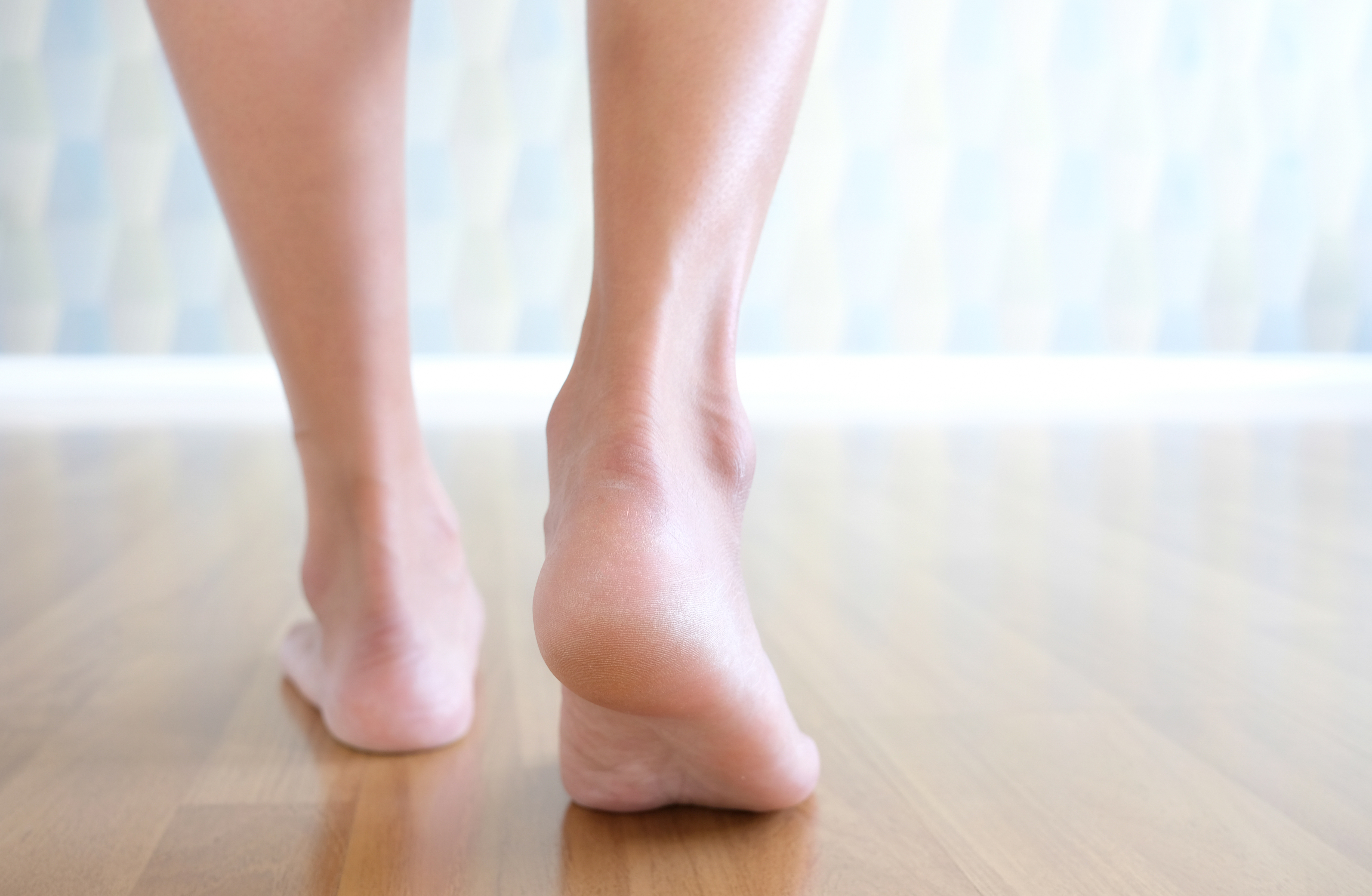
Our brain might come equipped with a noise-canceling feature: one that helps us ignore the sound of our own footsteps or the crunching of our bites.
In a new study, which was conducted in mice, the mouse brain canceled out the sound of its own footsteps. This ability helped the mice to better hear other sounds in their surroundings, researchers reported today (Sept. 12) in the journal Nature.
For a mouse walking around in a field, it's "better to hear a cat than its own footsteps," said senior study author Richard Mooney, a professor of neurobiology at Duke University. [3D Images: Exploring the Human Brain]
Mooney and his team used mice to study their "acoustic virtual reality system." They implanted tiny electrodes into their auditory cortex — the area of the brain that processes sound — and had the mice run on a treadmill under a microscope so that they could also take live images of the brain.
To see how the brain processed sounds associated with an animal's own movement, the researchers created artificial footstep sounds — sounds that mice wouldn't encounter in the wild. With each step the mice took, researchers played a quick note or a "tone pip." Just imagine the mice are running on a tiny piano, Mooney told Live Science. But "each key plays exactly the same note."
Mooney and his team found that after many thousands of footsteps over two to three days, activity in the auditory cortex decreased.
But when the researchers changed the sound of the pip, the auditory cortex became much more active. This could also explain why you can hear your footsteps if, say, you wear loud boots one day, and you don't typically, Mooney said.
Sign up for the Live Science daily newsletter now
Get the world’s most fascinating discoveries delivered straight to your inbox.
"Experience can shape how the brain suppresses predictable sensations that arise from movement," he said.
Their imaging and measurements showed a strong coupling between the motor cortex — an area of the brain that's involved with movement — and the auditory cortex. During training, the motor cortex begins forming synapses, or connections to the auditory cortex. These connections end up serving as a noise filter.
So-called inhibitory neurons, or brain cells, in the motor cortex began to send out signals to cancel out the firing of neurons in the auditory cortex that make us aware of the sound. This process is so quick that it is "predictive," Mooney said, meaning the cancellation signal happens at the same time that the brain commands a movement.
The researchers also found that mice that had been trained to ignore the sound of their own footsteps were better able to detect abnormal or new sounds when they were running, compared with those who hadn't gone through the training.
Mooney thinks the results could be very clearly translated to humans. Though the cortex is much more advanced in humans, "the basic brain architecture between the motor cortex and the auditory cortex is present in all mammals studied," he said.
"Mice don’t play the piano, at least none that I know do," Mooney said. For them, the ability to suppress movement-related sounds is more of a survival benefit, such as to better notice potential predators.
That may also be true for humans, but this auditory adaptation may also allow humans to partake in complex tasks like learning to talk, playing an instrument or singing, Mooney said.
This kind of system can train your brain to expect the notes you play or sing. "Once you've got a really good prediction of what should happen … you're also really sensitive to if it turns out different."
(Similar systems are known to exist in the human brain with movement: Take, for example, figure skaters. Their brains learn what movements to expect and begin to cancel out reflexes that would prevent their head-spinning twirls. But, if the figure skater makes a wrong landing, the brain considers that something unexpected and doesn't fire its inhibitory neurons — and fall-catching reflexes kick in.)
Further, understanding this system can be beneficial to studies on psychosis, according to Mooney. A common symptom of schizophrenia, for example, is voice-like hallucinations that are thought to be caused by a "broken" prediction circuit in the brain, he said. In other words, auditory brain cells aren't suppressed as much and fire too much, even when there are no external sounds to trigger them.
Originally published on Live Science.
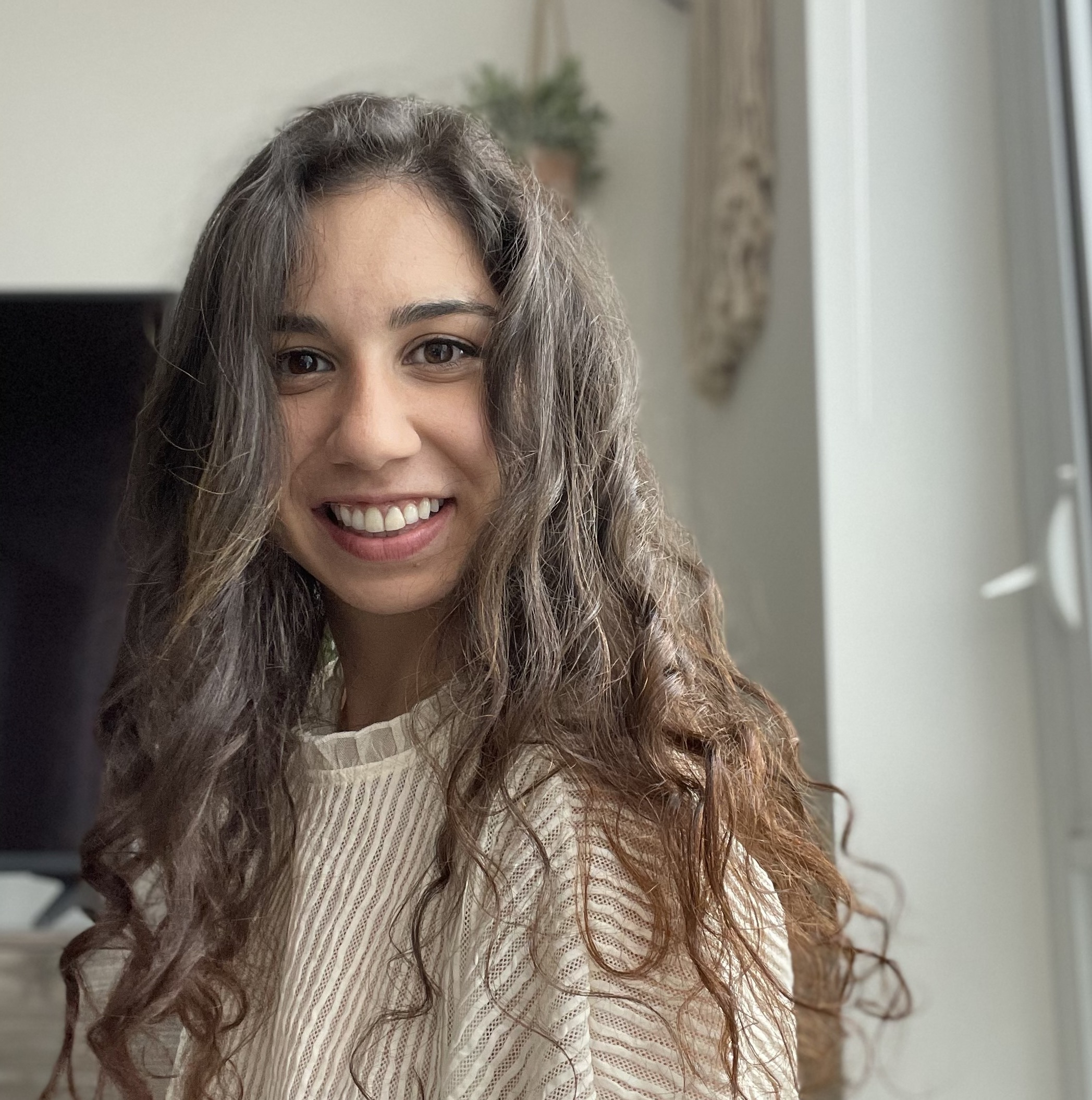
Yasemin is a staff writer at Live Science, covering health, neuroscience and biology. Her work has appeared in Scientific American, Science and the San Jose Mercury News. She has a bachelor's degree in biomedical engineering from the University of Connecticut and a graduate certificate in science communication from the University of California, Santa Cruz.