The Most Precise Measurement of Antimatter Yet Deepens the Mystery of Why We Exist
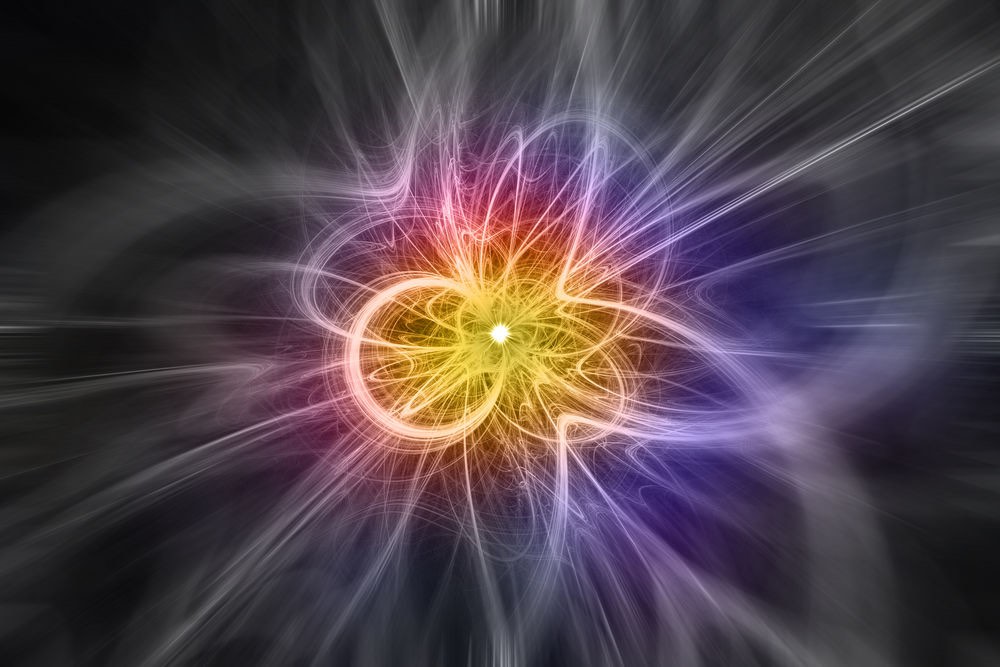
Scientists have made the most precise measurement of antimatter yet, and the results only deepen the mystery of why life, the universe, and everything in it exists.
The new measurements show that, to an incredibly high degree of precision, antimatter and matter behave identically.
Yet those new measurements can't answer one of the biggest questions in physics: Why, if equal parts matter and antimatter were formed during the Big Bang, is our universe today made up of matter?
Universe in balance
Our universe is predicated on the balance of opposites. For every type of "normal" particle, made of matter, there is a conjugate antiparticle of the same mass that has the opposite electric charge produced at the same time. Electrons have opposing antielectrons, or positrons; protons have antiprotons; and so on. [The 18 Biggest Unsolved Mysteries in Physics]
When matter and antimatter particles meet, however, they annihilate each other, leaving only leftover energy behind. Physicists posit that there should have been equal amounts of matter and antimatter created by the Big Bang, and each would have ensured the other's mutual destruction, leaving a baby universe bereft of life's building blocks (or anything, really). Yet here we are, in a universe made up almost wholly of matter.
But here's the kicker: We don't know of any primordial antimatter that made it out of the Big Bang. So why — if antimatter and matter behave the same way — did one type of matter survive the Big Bang and the other did not?
One of the best ways to answer that question is to measure the fundamental properties of matter and its antimatter conjugates as precisely as possible and compare those results, said Stefan Ulmer, a physicist at Riken in Wako, Japan, who was not involved in the new research. If there's a slight deviation between matter properties and correlated antimatter properties, that could be the first clue to solving physics' biggest whodunit. (In 2017, scientists found some slight differences in the way some matter antimatter partners behave, but the results weren't statistically strong enough to count as a discovery.)
Get the world’s most fascinating discoveries delivered straight to your inbox.
But if scientists want to manipulate antimatter, they have to painstakingly make it. In recent years, some physicists have taken to studying antihydrogen, or hydrogen's antimatter counterpart, because hydrogen is "oneof the things we understand best in the universe," study co-author Jeffrey Hangst, a physicist at Aarhus University in Denmark, told Live Science. Making antihydrogen typically involves mixing 90,000 antiprotons with 3 million positrons to produce 50,000 antihydrogen atoms, only 20 of which are caught with magnets in an 11-inch-long (28 centimeters) cylindrical tube for further study.
Now, in a new study published today (April 4) in the journal Nature, Hangst's team has achieved an unprecedented standard: They've taken the most precise measurement of antihydrogen — or any type of antimatter at all — to date. In 15,000 atoms of antihydrogen (think doing that aforementioned mixing process some 750 times), they studied the frequency of light the atoms emit or absorb when they jump from a lower energy state to a higher one. [Beyond Higgs: 5 Elusive Particles That May Lurk in the Universe]
The researchers' measurements showed that antihydrogen atoms' energy levels, and the amount of light absorbed, agreed with their hydrogen counterparts, with a precision of 2 parts per trillion, dramatically improving upon the previous measurement precision on the order of parts per billion.
"It's very rare that experimentalists manage to increase precision by factor of 100," Ulmer told Live Science. He thinks that, if Hangst's team continues the work for an additional 10 to 20 years, they will be able to increase their level of hydrogen spectroscopy precision by a further factor of 1,000.
For Hangst — the spokesperson for the ALPHA collaboration at the European Organization for Nuclear Research (CERN), which produced these results — this achievement was decades in the making.
Trapping and holding antimatter was a major feat, Hangst said.
"Twenty years ago, people thought this would never happen," he said. "It's an experimental tour de force to be able to do this at all."
The new results are very impressive, Michael Doser, a physicist at CERN who was not involved in the work, told Live Science in an email.
"The number of trapped atoms for this measurement (15,000) is a huge improvement on [Hangst's group's] own records of only a few years ago," Doser said.
So what does the most precise measurement of antimatter even tell us? Well, unfortunately, not much more than we already knew. As expected, hydrogen and antihydrogen — matter and antimatter — behave identically. Now, we just know that they're identical at a measurement of parts per trillion. However, Ulmer said the 2-parts-per-trillion measurement does not rule out the possibility that something is deviating between the two types of matter at an even greater level of precision that has thus far defied measurement.
As for Hangst, he's less concerned with answering the question of why our universe of matter exists as it does without antimatter — what he calls "the elephant in the room." Instead, he and his group want to focus on making even more precise measurements, and exploring how antimatter reacts with gravity — does it fall down like normal matter, or could it fall up?
And Hangst thinks that mystery could be solved before the end of 2018, when CERN will shut down for two years for upgrades. "We have other tricks up our sleeve," he said. "Stay tuned."
Original article on Live Science.
Aylin Woodward is a science reporter who covers space exploration, anthropology, paleontology, physics and material sciences. She has written for Business Insider and now reports at The Wall Street Journal. She graduated from the University of California, Santa Cruz science communication Master's program, and earned a bachelor's degree from Dartmouth College. She received a National Science Foundation Graduate Research Fellowship in 2016 for work focused on hominin bipedalism.