'Holy Grail' Hadron: Scientists Are Close to Detecting the Elusive Tetraquark Particle
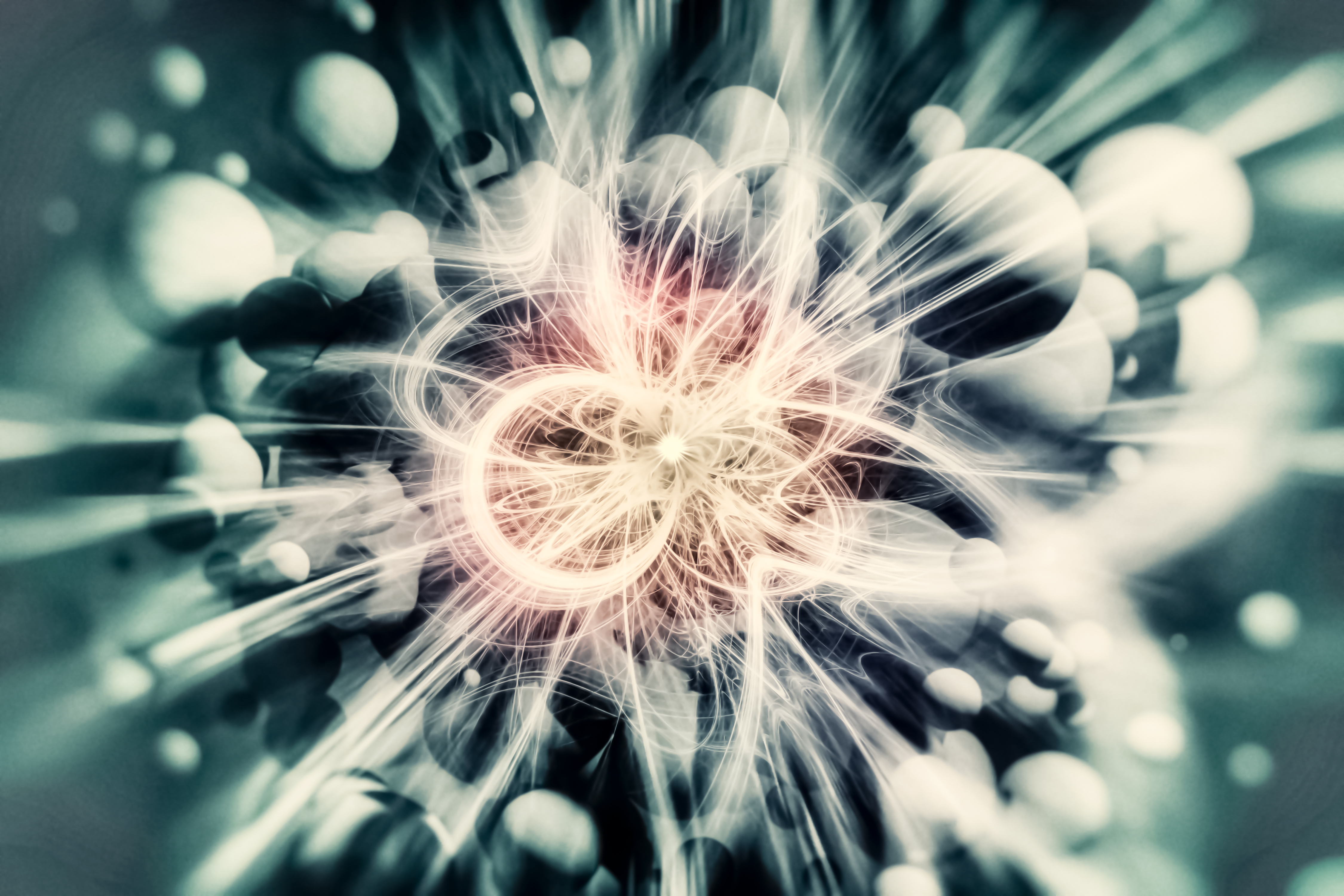
Flit, zip, jitter, boom. Quarks, the tiny particles that make up everything tangible in the universe, remain deeply mysterious to physicists even 53 years after scientists first began to suspect these particles exist. They bop around at the edge of scientific instruments' sensitivities, are squirreled away inside larger particles, and decay from their higher forms into their simplest in half the time it takes a beam of light to cross a grain of salt. The little buggers don't give up their secrets easily.
That's why it took more than five decades for physicists to confirm the existence of an exotic particle they've been hunting since the beginning of quark science: the massive (at least in subatomic particle terms), elusive tetraquark.
Physicists Marek Karliner of Tel Aviv University and Jonathan Rosner of the University of Chicago have confirmed that the strange, massive tetraquark can exist in its purest, truest form: four particles, all interacting with one another inside a single, larger particle, with no barriers keeping them apart. It's stable, they found, and can likely be generated at the Large Hadron Collider, a particle smasher at the CERN particle physics laboratory in Switzerland, they report in a paper to be published in a forthcoming issue of the journal Physical Review Letters. [Beyond Higgs: 5 Elusive Particles That May Lurk in the Universe]
Hold up — what the quark is a quark?
If you know a little about particle physics, you probably know that everything with mass is made up of atoms. Diving a little deeper into particle physics would reveal that those atoms are made up of subatomic particles — protons, neutrons and electrons. An even deeper look would reveal quarks.
Neutrons and protons are the most common examples of a class of particles known as hadrons. If you could peer into a hadron, you'd find it's made up of even more basic particles, clinging tightly together. Those are quarks.
Like atoms, which adopt different properties depending on the combinations of protons and neutrons in their nuclei, hadrons derive their properties from combinations of their resident quarks. A proton? That's two "up" quarks and one "down" quark. Neutrons? Those are made up of two "down" quarks and one "up" quark. [Wacky Physics: The Coolest Little Particles in Nature]
(Electrons aren't made up of quarks because they aren't hadrons — they're leptons, part of a class of distant cousins of quarks.)
Get the world’s most fascinating discoveries delivered straight to your inbox.
"Up" and "down" are the most common flavors of quark, but they're just two out of six. The other four — "charm," "top," "strange" and "bottom" quarks — existed in the moments after the Big Bang, and they appear in extreme situations, such as during high-velocity collisions in particle colliders. But they're much heavier than up and down quarks, and they tend to decay into their lighter siblings within moments of their creation.
But those heavier quarks can last long enough to bind together into strange hadrons with unusual properties that are stable for the very short lifetimes of the quarks zipping around inside them. Some good examples: the "doubly charmed baryon," or a hadron made up of two charm quarks and a lighter quark; and its cousin, formed when a hadron made up of two bulky bottom quarks and one lighter quark fuse together in a flash more powerful than the individual fusion reactions inside hydrogen bombs. (Of note, the bottom quark fusion is militarily useless thanks to heavy quarks' short lifetimes.)
Playing with colors
"The suspicion had been for many years that [the tetraquark] is impossible," Karliner told Live Science.
That's because physical laws suggested four quarks couldn't actually bind together into a stable hadron. Here's why: Just like in atoms, where the attraction between positively charged protons and negatively charged electrons is what holds them together, hadrons are held together by forces as well. In atoms, positive and negative particles constantly try to neutralize their charges to zero, so protons and electrons stick together, canceling each other out. [7 Strange Facts About Quarks]
Quarks have positive and negative electrodynamic charges, but they also interact with one another via the much more powerful "strong" force. And the strong force also has charges, called color charges: red, green and blue.
Any quark can have any color charge. And when they bind together to form hadrons, all those charges have to cancel out. So a red quark, for example, has to hook up with either a green quark and a blue quark, or its antimatter twin — an "antiquark" with a color charge of "antired." (This is your brain on quantum mechanics.) Any combination of a color and its anticolor, or all three colors, sticking together has a neutral color charge. Physicists call these particles "white."
The tetraquark: It's like a relationship (in that it doesn't always work)
So, Karliner said, it's not hard to imagine a four-quark hadron: Just stick two quarks to two matching antiquarks. But just because you stick four matching quarks together, he said, doesn't mean they'll be stable enough to form an actual hadron — they could fly apart.
"Just because you move two men and two women into an apartment," Karliner said, "doesn't mean they'll settle down and form a nuclear family."
Quarks have mass, which physicists measure in units of energy: megaelectron volts, or MeV. When they bind together, some of that mass converts into the binding energy holding them together, also measured in MeV. (Remember Einstein's E=mc^2? That's energy equals mass-times-the-speed-of-light-squared, the equation governing that conversion.)
If the mass is too high compared with the binding force, the energy of the quarks careening around inside the hadron will tear the particle apart. If it's low enough, the particle will live long enough for the quarks to settle down and develop group properties before they decay. A big, happy quark-foursome family needs to have a mass lower than two mesons (or quark-antiquark pairs) stuck together, according to Karliner.
Unfortunately, the mass of a quark family after some of its bulk is converted into binding force is incredibly difficult to calculate, which makes it hard to figure out whether a given theoretical particle is stable.
Scientists have known for about a decade that mesons can bind to other mesons to form ad-hoc tetraquarks, which is why you might have seen reports touting the existence of tetraquarks before. But in those tetraquarks, each quark interacts primarily with its pair. In a true tetraquark, all four would mix with one another equally.
"It's charming and interesting, but not the same," Karliner said. "It's very different to have two couples in different rooms sharing an apartment, and two men and two women all together with everyone … interacting with everyone else."
But those double-meson tetraquarks provide the mass threshold that true tetraquarks must cross to be stable, he said.
A needle in a haystack of haystacks
In theory, Karliner said, it would be possible to predict the existence of a stable tetraquark from pure calculation. But the quantum mechanics involved were just too difficult to make work with any reasonable degree of confidence.
Karliner and Rosner's key insight was that you could start to figure out the mass and binding energy of rare hadrons by analogy to more common hadrons that had already been measured.
Remember that doubly charmed baryon from earlier? And its explosive cousin with the two bottom quarks? In 2013, Karliner and Rosner began to suspect they could calculate its mass, after thinking carefully about the binding energy inside mesons made up of charm quarks and anticharm quarks.
Quantum mechanics suggests that two different-colored charm quarks — say, a red charm and a green charm — should bind together with exactly half the energy of a charm quark and its antimatter twin — say, a red charm quark and an antired charm antiquark. And scientists have already measured the energy of that bond, so the energy of acharm-charm bond should be half of that.
So Karliner and Rosner worked with those numbers, and they found that the doubly charmed baryon and double-bottom baryon should have a mass of 3627 MeV, plus or minus 12 MeV. They published their papers and pushed the experimentalists at CERN (European Organization for Nuclear Research) to start hunting, Karliner said.
But Karliner and Rosner offered CERN a road map, and eventually, the CERN scientists acceded. In July 2017, the first definite doubly charmed baryons turned up in the Large Hadron Collider (LHC). [Photos: The World's Largest Atom Smasher (LHC)]"The experimentalists were quite skeptical at first" that it would be possible to find the doubly charmed baryons in the real world, Karliner said. "It's like looking for a needle not in a haystack, but in a haystack of haystacks."
"We predicted in 2014 that the mass of this doubly charmed baryon was going to be 3,627 MeV, give or take 12 MeV," Karliner said. "The LHC measured 3,621 MeV, give or take 1 MeV."
In other words, they nailed it.
And because their calculation turned out to be correct, Karliner and Rosner had a road map to the true stable tetraquark.
One big, fat, happy family
In quantum mechanics, Karliner explained, there's a general rule that heavier quarks tend to bind much more tightly to each other than lighter quarks do. So if you're going to find a stable tetraquark, it's probably going to involve some quarks from the heavier end of the flavor spectrum.
Karliner and Rosner got to work as soon as the doubly charmed baryon measurement was announced. First, they calculated the mass of a tetraquark made up of two charm quarks and two lighter antiquarks; charm quarks, after all, are pretty chunky, at about 1.5 times the mass of a proton. The result? A doubly-charmed tetraquark turns out to be right on the edge of stable and unstable, with room for error on both sides — in other words, too uncertain to call a discovery.
But charm quarks aren't the heaviest quarks around. Enter the bottom quark, a true monster of an elementary particle at about 3.5 times the mass of its charmed sibling, with an accompanying leap in binding energy.
Fuse two of those together, Karliner and Rosner calculated, along with an up antiquark and a down antiquark, and you'll end up with a stable foursome — converting so much of their bulk into binding energy that they end up 215 MeV under the maximum mass threshold, with a margin of error of just 12 MeV.
"The upshot of all this is that we now have a robust prediction for the mass of this object which had been the holy grail of this branch of theoretical physics," Karliner said.
This kind of tetraquark won't live very long once it's created; it winks out after just one-tenth of a picosecond, or the length of time it takes a beam of light to cross a single microscopic skin cell. It then will decay into simpler combinations of up and down quarks. But that 0.1 picoseconds (one ten-trillionth of a second) is plenty long enough on the quantum mechanical scale to be considered a stable particle.
"It's like if you compared a human lifetime to [the movement of continents]," Karliner said. "If you have some creatures living on the scale of fractions of seconds, a human lifetime would seem almost infinite."
Onward to Switzerland
The next step, once a particle has been predicted by theorists, is for the experimentalists at CERN to try to create it in the miles-long tubes of their particle smasher, the LHC.
That can be a grueling process, especially because of the specific properties of bottom quarks.
The LHC works by slamming protons together at large fractions of the speed of light, releasing enough energy into the collider that some of it turns back into mass. And some tiny fraction of that mass will condense into rare forms of matter — like that doubly charmed baryon.
But the heavier a particle is, the lower the odds it will pop into being in the LHC. And bottom quarks are exceptionally unlikely creations.
In order to build a tetraquark, Karliner said, the LHC has to generate two bottom quarks in close enough proximity to each other that they bind, and then "decorate" them with two light antiquarks. And then it has to do it again, and again — until it's happened enough times that the researchers can be sure of their results.
But that's not as unlikely as it may sound.
"It turns out that, if you consider how you would make such things in a lab," Karliner said, "the probability of making them is only slightly less likely than finding that baryon with two bottom quarks and one light quark."
And that hunt is already underway.
Once the two-bottom-quark baryon is discovered, Karliner said — a result he expects within the next few years — "the clock starts ticking" on the appearance of the tetraquark.
Somewhere out there in the ether is a hadron that physicists have been hunting for 53 years. But now they've caught its scent.
Editor's Note: This article was updated to correct a the mass of the researcher's earlier doubly-charmed baryon prediction. It was 3,627 MeV, not 4,627 MeV.
Originally published on Live Science.
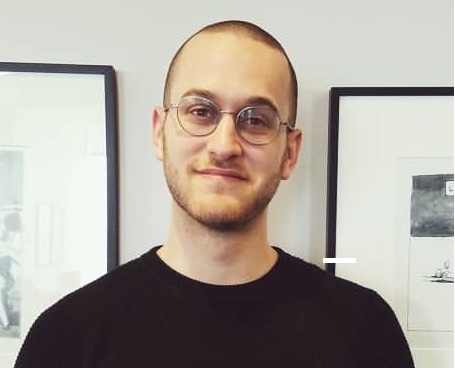