Looking for a Change: Cell Motility Crucial for Development
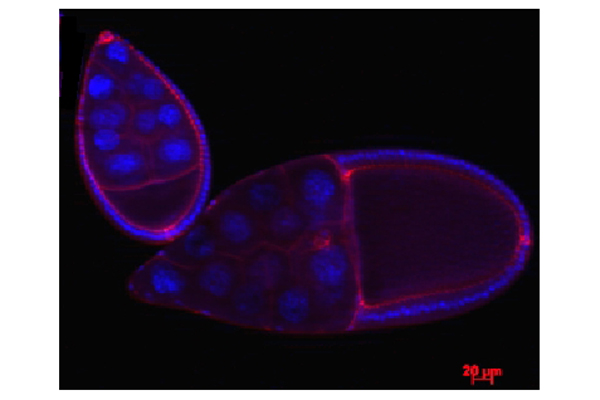
This Behind the Scenes article was provided to LiveScience in partnership with the National Science Foundation.
Cells are constantly migrating throughout our bodies. White blood cells rush to a site of infection. Skin cells rally to repair a wound. And when one is afflicted with cancer, those cells metastasize and travel to distant organs. For a cell to detach from its original place and travel, it must change its identity — a matter of turning on new genes for expression. To predict which genes regulate cell motility in humans, Michelle Starz-Gaiano, a developmental biologist at the University of Maryland, Baltimore County, has turned to an insect whose genes are at least 70 percent similar to ours — the fruit fly.
When I visited her lab to learn more about her work, Starz-Gaiano's enthusiasm for her subject was clear from the start of our interview. "Cell migration is required for an egg to grow into a beautiful organism. So understanding how any cell moves is crucial for understanding development," she said. "It's fascinating to think about how cells can do things that I, as a very complex organism, have trouble doing, like finding direction and being on time," she said with a laugh.
With funding from the National Science Foundation and the March of Dimes, Starz-Gaiano studies how cells move within fruit fly egg chambers. When the fruit fly egg prepares itself for fertilization, some cells, to do their jobs, must travel to new sites. Starz-Gaiano focuses on the cells whose task is to build a "door" for the sperm's grand entrance. Fertilization depends on the success of their migration and the door's formation.
Front Row Seats
Starz-Gaiano's work on cell motility during egg development started at the Johns Hopkins University School of Medicine with Denise Montell, director of the Center for Cell Dynamics. They chose fruit flies as a model because of the tremendous number of genetic-research tools available from a century of fruit fly research. Because the insect's tissues are transparent, the scientists could also take advantage of front row seats to molecular scenes.
"A lot of what we knew about cell migration came from watching cells move in a dish. But that didn't tell us the relationship between tissues and individual cells," said Starz-Gaiano. So Montell's group worked out a way to watch the dramatic migration using time-lapse imaging, which produced a 10-second movie of what, in real-time, would be a two-hour event. A combination of fruit fly egg cells moving in their normal environment and the live-imaging method revealed the complexities of a scale of life invisible to the naked eye.
Sign up for the Live Science daily newsletter now
Get the world’s most fascinating discoveries delivered straight to your inbox.
Starz-Gaiano tucked her short blonde hair behind her ear and sat on the edge of her chair, ready to get technical. "Can I show you some PowerPoint slides?" she asked. "It's hard to just explain in words." With pictures, diagrams and a time-lapse video, she unraveled stages eight through ten of the developing fruit fly egg.
Suspended in blackness is a delicate teardrop of cells. Half the egg is the oocyte, the single cell designated to divide and grow into an offspring. The other half is composed of 15 nurse cells whose role is to look after the egg's development. About 600 to 900 epithelial cells, regular in shape, stiff, and tightly connected to each other, pad the outer wall. They eventually will form the eggshell.
Rock Climbers
From the eggshell group, two polar cells and six to eight of their neighbors are about to change their fates. They will cluster, journey to the oocyte and build the small door for the sperm.
The polar cells have no moving ability, so they must recruit the surrounding six to eight cells to border them and carry them away. When it's time to go, the pair sends a signal, a cytokine-like protein, to their neighbors. An elaborate signaling pathway takes place inside the recipients to express the slbo (pronounced slow-bo) gene. And voila, the nearby epithelial cells begin their new life as border cells.
They tightly encase the two polar cells like bodyguards. The rock climbers travel in unison, lunging and latching onto one molecular grip after another. A Velcro-like protein allows them to stick and release from the highway of filaments. The blob rotates and cells take turns leading. They work together, unrelenting, shouldering through nurse cells along the way. Anything can go wrong across the microscopic terrain. Yet they almost always make it.
Years of lab work allow Starz-Gaiano and her colleagues to begin to understand the signaling pathways that regulate this series of events. "It's interesting how individual molecules can control complex behaviors. Migration has to be really tightly regulated to work out, and it works out so much of the time."
Signaling Pathway
Among the molecular checks and balances is what's called a JAK/STAT signaling pathway. When a nearby cell receives the first signal from the polar cell, two enzymes named JAKs, or Janus kinases, wake up. Then the JAKs call over two STAT molecules, short for Signal Transducer and Activator of Transcription. Once the STATs pair up, their job is to find the DNA and target the dormant slbo gene for expression. In this way, JAK/STAT controls the activation of motility in border cells.
The JAK/STAT signaling cascade is well known in mammals, particularly for its role in hematopoiesis--the process of stem cells becoming blood cells. In addition, high STAT activity has been correlated with more invasive cancers, such as ovarian cancer.
When Dr. Starz-Gaiano's UMBC lab delved into what regulates STAT, they focused on two different genes found in border cells. One is the apontic gene, which shuts off STAT activity below a certain threshold, stopping extra cells from tagging along and slowing down the group. The second is the socs36E gene, which stunts movement by suppressing the cytokine signaling.
"We are able to study the fruit fly genes through loss-of-function experiments," she explained. "By blocking one gene and watching what goes wrong, we can understand its role in development. For example, if you bake bread and leave out the yeast, the bread doesn't rise. You can conclude that the yeast's job is to make the bread rise. We did the same thing with genes and proteins in flies."
Lab Work
She led me out of her office and to the labs, where a handful of undergraduate and graduate students toiled away under her guidance. "Salma wants to know how we do anything around here!" she announced, smiling.
Katie, the lab technician, sat on a bench peering into a microscope. Her ear buds drowned out the loud mechanical humming in the room with music. She was inspecting fruit flies knocked unconscious by carbon dioxide pumped into the pad they lay on. While they slept, she could pick out the unmated females. A poster on the opposite wall served as a guide. Rows of flies modeled different physical characteristics, the bottom row indicating what males and females look like.
Starz-Gaiano opened incubators to show me shelves filled with clear vials neatly labeled. Inside each swarmed flies of all ages, from tiny larvae to adults. They live on cornmeal and molasses. "We grow little families with the same mutations. Katie flips the vials once a month into new food." Each mutant line, such as one with no STAT activity, has to be raised in a separate vial.
Jinal, an undergraduate student, yanked out the female ovaries with a set of forceps and dropped them into a tube of liquid. A durable exoskeleton kept the flies' bodies intact. Later, the cells of interest would be fluorescently marked.
After all the sorting, dissecting and staining came the fun part — examining the eggs with a compound epifluorescent microscope. The enormous machine includes a component that allows the viewer to look at a thick tissues one cross-section at a time. It also enables live imaging. On the adjacent computer screen, Starz-Gaiano clicked around and brought up beautiful shots of egg chambers, each indicated by their glowing neon colors.
The professor pointed out how her lab wasn't just a lab, it was a workplace. "The students in my lab are being trained through the NSF grant. People don't always realize that funding not only provides for scientific research, but it also creates jobs for a lot of people."
Like the cluster of polar and border cells, the lab's sense of direction was acute, their teamwork admirable. They moved with a common goal to explore fundamental questions about how cells travel, to better understand the human immune system, stem cells and even cancer.
Starz-Gaiano showed me one last photograph, marveling at the way cells in a developing egg could be extremely sticky, but travel with agility. Despite the answers they had found so far, there was still much to learn about the smallest unit of life. "How do cells navigate a constantly changing environment? How do they understand what time to go and where to go?" she wondered aloud. "It's just, I think, a miracle."
Editor's Note: The researchers depicted in Behind the Scenes articles have been supported by the National Science Foundation, the federal agency charged with funding basic research and education across all fields of science and engineering. Any opinions, findings, and conclusions or recommendations expressed in this material are those of the author and do not necessarily reflect the views of the National Science Foundation. See the Behind the Scenes Archive.