What is a superconductor?
In a superconductor, an electric current can exist forever.
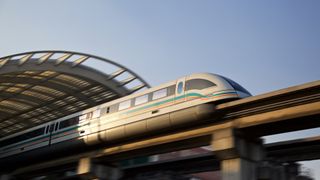
A superconductor is a material that achieves superconductivity, which is a state of matter that has no electrical resistance and does not allow magnetic fields to penetrate. An electric current in a superconductor can persist indefinitely.
Superconductivity can only typically be achieved at very cold temperatures. Superconductors have a wide variety of everyday applications, from MRI machines to super-fast maglev trains that use magnets to levitate the trains off the track to reduce friction. Researchers are now trying to find and develop superconductors that work at higher temperatures, which would revolutionize energy transport and storage.
Who discovered superconductivity?
The credit for the discovery of superconductivity goes to Dutch physicist Heike Kamerlingh Onnes. In 1911, Onnes was studying the electrical properties of mercury in his laboratory at Leiden University in The Netherlands when he found that the electrical resistance in the mercury completely vanished when he dropped the temperature to below 4.2 Kelvin — that's just 4.2 degrees Celsius (7.56 degrees Fahrenheit) above absolute zero.
To confirm this result, Onnes applied an electric current to a sample of supercooled mercury, then disconnected the battery. He found that the electric current persisted in the mercury without decreasing, confirming the lack of electrical resistance and opening the door to future applications of superconductivity.
History of superconductivity
Physicists spent decades trying to understand the nature of superconductivity and what caused it. They found that many elements and materials, but not all, become superconducting when cooled below a certain critical temperature.
In 1933, physicists Walther Meissner and Robert Ochsenfeld discovered that superconductors "expel" any nearby magnetic fields, meaning weak magnetic fields can't penetrate far inside a superconductor, according to Hyper Physics, an educational site from the Georgia State University department of physics and astronomy. This phenomenon is called the Meissner effect.
It wasn't until 1950 that theoretical physicists Lev Landau and Vitaly Ginzburg published a theory of how superconductors work, according to Ginzburg's biography on The Nobel Prize website. While successful in predicting the properties of superconductors, their theory was "macroscopic," meaning it focused on the large-scale behaviors of superconductors while remaining ignorant of what was going on at a microscopic level.
Sign up for the Live Science daily newsletter now
Get the world’s most fascinating discoveries delivered straight to your inbox.
Finally, in 1957, physicists John Bardeen, Leon N. Cooper and Robert Schrieffer developed a complete, microscopic theory of superconductivity. To create electrical resistance, the electrons in a metal need to be free to bounce around. But when the electrons inside a metal become incredibly cold, they can pair up, preventing them from bouncing around. These electron pairs, called Cooper pairs, are very stable at low temperatures, and with no electrons "free" to bounce around, the electrical resistance disappears. Bardeen, Cooper and Schrieffer put these pieces together to form their theory, known as BCS theory, which they published in the journal Physical Review Letters.
How do superconductors work?
When a metal drops below a critical temperature, the electrons in the metal form bonds called Cooper pairs. Locked up like this, the electrons can't provide any electrical resistance, and electricity can flow through the metal perfectly, according to the University of Cambridge.
However, this only works at low temperatures. When the metal gets too warm, the electrons have enough energy to break the bonds of the Cooper pairs and go back to offering resistance. That is why Onnes, in his original experiments, found that mercury behaved as a superconductor at 4.19 K, but not 4.2 K.
What are superconductors used for?
It's very likely that you've encountered a superconductor without realizing it. In order to generate the strong magnetic fields used in magnetic resonance imaging (MRI) and nuclear magnetic resonance imaging (NMRI), the machines use powerful electromagnets, as described by the Mayo Clinic. These powerful electromagnets would melt normal metals due to the heat of even a little bit of resistance. However, because superconductors have no electrical resistance, no heat is generated, and the electromagnets can generate the necessary magnetic fields.
Similar superconducting electromagnets are also used in maglev trains, experimental nuclear fusion reactors and high-energy particle accelerator laboratories.Superconductors are also used to power railguns and coilguns, cell phone base stations, fast digital circuits and particle detectors.
Essentially, any time you need a really strong magnetic field or electric current and don't want your equipment to melt the moment you turn it on, you need a superconductor.
"One of the most interesting applications of superconductors is for quantum computers," said Alexey Bezryadin, a condensed matter physicist at the University of Illinois at Urbana-Champaign. Because of the unique properties of electrical currents in superconductors, they can be used to construct quantum computers.
"Such computers are composed of quantum bits or qubits. Qubits, unlike classical bits of information, can exist in quantum superposition states of being '0' and '1' at the same time. Superconducting devices can mimic this," Bezryadin told Live Science. "For example, the current in a superconducting loop can flow clockwise and counterclockwise at the same time. Such a state constitutes an example of a superconducting qubit."
What's the latest in superconductor research?
The first challenge for today's researchers is "to develop materials that are superconductors at ambient conditions, because currently superconductivity only exists either at very low temperatures or at very high pressures," said Mehmet Dogan, a postdoctoral researcher at the University of California, Berkeley. The next challenge is to develop a theory that explains how the novel superconductors work and predict the properties of those materials, Dogan told Live Science in an email.
Superconductors are separated into two main categories: low-temperature superconductors (LTS), also known as conventional superconductors, and high-temperature superconductors (HTS), or unconventional superconductors. LTS can be described by the BCS theory to explain how the electrons form Cooper pairs, while HTS use other microscopic methods to achieve zero resistance. The origins of HTS are one of the major unsolved problems of modern-day physics.
Most of the historical research on superconductivity has been in the direction of LTS, because those superconductors are much easier to discover and study, and almost all applications of superconductivity involve LTS.
HTS, in contrast, are an active and exciting area of modern-day research. Anything that works as a superconductor above 70 K is generally considered an HTS. Even though that's still pretty cold, that temperature is desirable because it can be reached by cooling with liquid nitrogen, which is far more common and readily available than the liquid helium needed to cool to the even lower temperatures that are needed for LTS.
The future of superconductors
The "holy grail" of superconductor research is to find a material that can act as a superconductor at room temperatures. To date, the highest superconducting temperature was reached with extremely pressurized carbonaceous sulfur hydride, which reached superconductivity at 59 F (15 C, or about 288 K), but required 267 gigapascals of pressure to do it. That pressure is equivalent to the interior of giant planets like Jupiter, which makes it impractical for everyday applications.
Room-temperature superconductors would allow for the electrical transmission of energy with no losses or waste, more efficient maglev trains, and cheaper and more ubiquitous use of MRI technology. The practical applications of room-temperature superconductors are limitless — physicists just need to figure out how superconductors work at room temperatures and what the "Goldilocks" material to allow for superconductivity might be.
Additional resources
- Watch "The Map of Superconductivity" from Domain of Science on YouTube.
- Read "Superconductivity: A Very Short Introduction" (Oxford University Press, 2005) by Stephen J. Blundell.
- Learn more about superconductors at superconductors.org.
Paul M. Sutter is a research professor in astrophysics at SUNY Stony Brook University and the Flatiron Institute in New York City. He regularly appears on TV and podcasts, including "Ask a Spaceman." He is the author of two books, "Your Place in the Universe" and "How to Die in Space," and is a regular contributor to Space.com, Live Science, and more. Paul received his PhD in Physics from the University of Illinois at Urbana-Champaign in 2011, and spent three years at the Paris Institute of Astrophysics, followed by a research fellowship in Trieste, Italy.