After the 'Great Dying,' life on Earth took millions of years to recover. Now, scientists know why.
Microorganisms might explain the slow rebound from the "Great Dying."
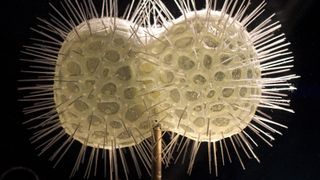
At the end of the Permian period 252 million years ago, Earth was devastated by a mass extinction that exterminated more than 90% of species on the planet. Compared with other mass extinctions, recovery from the "Great Dying" was slow: It took at least 10 million years for the planet to be repopulated and begin to restore its diversity.
Now, scientists might have figured out what delayed Earth's recovery. A group of tiny marine organisms called radiolarians vanished in the extinction's aftermath. Their absence radically altered marine geochemistry, enabling a type of clay formation that released carbon dioxide. This carbon dioxide release would have kept the atmosphere warm and the oceans acidic, thereby slowing the rebound of life, the scientists explained in a paper published Oct. 3 in the journal Nature Geoscience.
These were extreme conditions that hadn't been seen on Earth for hundreds of millions of years, before the advent of widespread life, study co-author Clément Bataille, now a professor of Earth and environmental sciences at the University of Ottawa in Canada, told Live Science.
"It just shows how much we don't know about these biogeochemical cycles and how a little change can really throw the system out of balance very quickly," Bataille said.
An unfriendly Earth
Bataille worked on the research as a postdoctoral scholar in the laboratory of Xiao-Ming Liu, a geochemist at the University of North Carolina at Chapel Hill. The researchers were trying to understand changes in Earth's climate at the end of the Permian (298.9 million to 251.9 million years ago) and the beginning of the Triassic (251.9 million to 201.3 million years ago). At the time, all of the continents were joined into one massive landmass called Pangaea, and a huge block of volcanoes known as the Siberian Traps were belching out planet-warming greenhouse gases, probably contributing to the extinction event that resulted in the death of almost everything.
The team wanted to study a process called chemical weathering — when rocks on land break down and release calcium, which erodes into the oceans. There, the calcium combines with carbon dioxide (CO2) to form carbonate rocks. The warmer the climate, the faster weathering occurs, because chemical reactions happen faster in warmer temperatures and more flowing water means more erosion. This creates a feedback loop that keeps global temperatures in check, Bataille said: When it's warmer and weathering is faster, more CO2 flows into the sea and gets locked up in ocean rocks, helping to cool the climate. When the climate cools, weathering slows and less CO2 is locked up in ocean rocks, thus preventing things from getting too chilly.
But there's another process that can occur in the ocean, called reverse weathering. This happens when the mineral silica is abundant and forms new clays on the ocean floor. During reverse weathering, these clays release more CO2 than carbonate rocks can capture.
Sign up for the Live Science daily newsletter now
Get the world’s most fascinating discoveries delivered straight to your inbox.
Silica isn't abundant in today's oceans because tiny planktonic organisms snatch it up to make their shells, so reverse weathering doesn't happen much. Similarly, in the Permian, tiny organisms called radiolarians took up almost all of the silica, thus keeping reverse weathering to a minimum.
A sudden shift
All of that may have changed, however, at the end of the Permian and the beginning of the Triassic. At this point, silica-rich rocks made of countless radiolarian shells disappeared, indicating that the radiolarians may have been snuffed out. At the same time, the balance of certain variants of molecules in ocean rocks went haywire, Bataille, Liu and their colleagues found.
The researchers were studying ratios of isotopes of lithium. Isotopes are versions of an element with slightly different atomic weights than the norm because they have different numbers of neutrons in their nuclei. Because of their different weights, various lithium isotopes are taken up in different ratios when new clays are formed, which happens in reverse weathering. The researchers found that some lithium isotopes virtually disappeared from the ocean right before the Great Dying and didn't recover for some 5 million years into the Triassic. This paints a picture of a world where the loss of radiolarians led to an ocean chock-full of silica, thus allowing reverse weathering to occur, Bataille said. The CO2 released by reverse weathering could have overwhelmed the CO2-trapping chemical weathering happening at the time and, in turn, kept the climate extra-steamy. Under such conditions, life would have struggled.
This is the first direct evidence that reverse weathering was happening at this time, said Hana Jurikova, a marine biogeochemist at the University of St. Andrews in Scotland. Jurikova was not involved in the research, but she wrote an editorial accompanying the paper in the journal Nature Geoscience.
"There's obviously a lot more work to be done," Jurikova told Live Science, "but it's kind of an elegant theory."
Among the questions yet to be answered is, what killed the radiolarians? Evidence shows that the reverse weathering began a few million years before the mass extinction, Jurikova said, suggesting that perhaps these microorganisms were already struggling before the Siberian Traps did their worst. Perhaps conditions were becoming challenging for life even before the life-extinguishing volcanic eruptions.
"We've been traditionally really excited about the mass extinction and trying to zoom in as much as we can," Jurikova said, "but maybe we're finding that we have to zoom out."
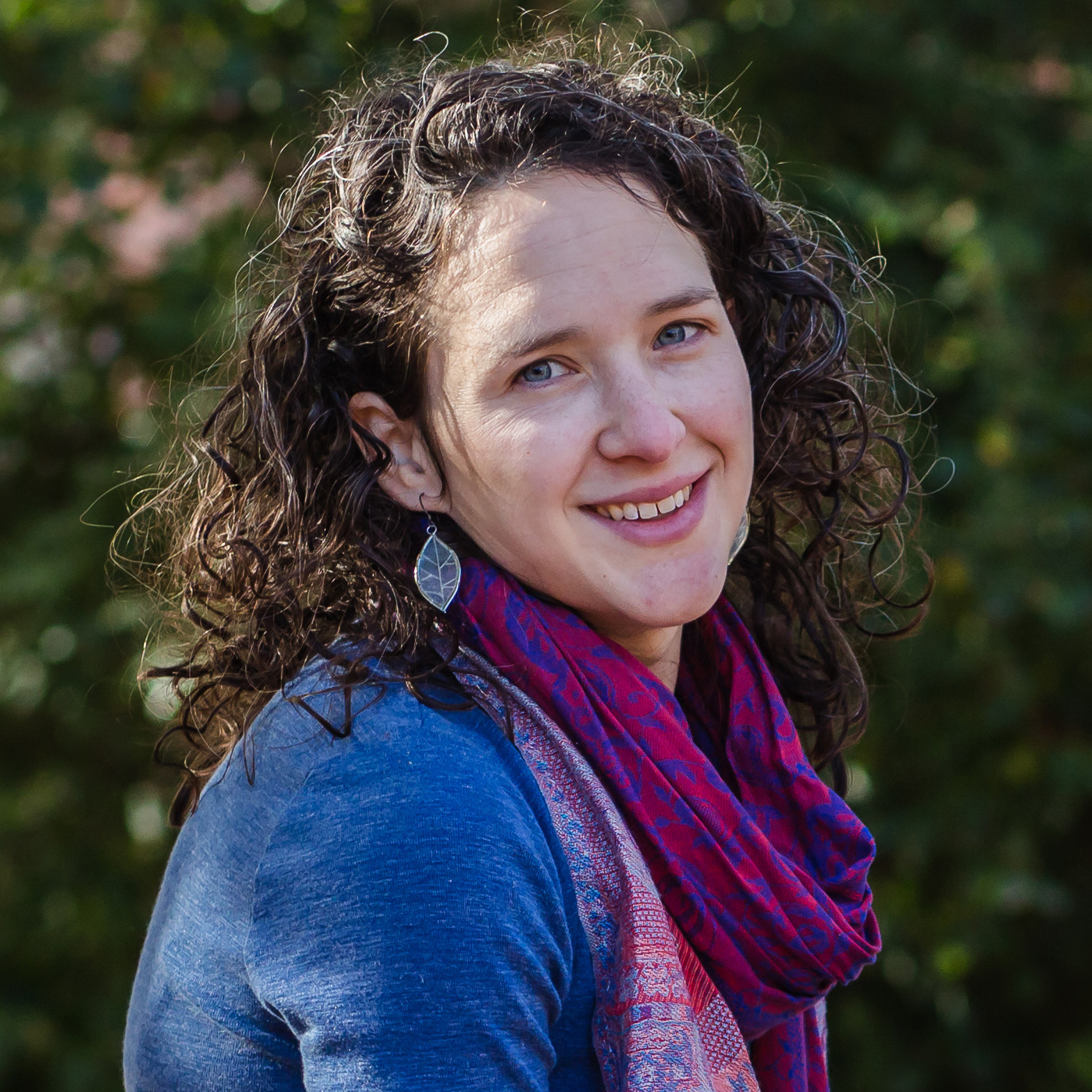
Stephanie Pappas is a contributing writer for Live Science, covering topics ranging from geoscience to archaeology to the human brain and behavior. She was previously a senior writer for Live Science but is now a freelancer based in Denver, Colorado, and regularly contributes to Scientific American and The Monitor, the monthly magazine of the American Psychological Association. Stephanie received a bachelor's degree in psychology from the University of South Carolina and a graduate certificate in science communication from the University of California, Santa Cruz.