Elusive 'Buchdahl stars' are black holes without event horizons. But do they really exist?
These hypothetical stars are the densest objects in the universe that can exist without becoming full-fledged black holes.
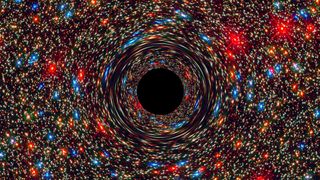
An elusive object in space has posed a riddle for scientists. It looks like a black hole. It acts like a black hole. It may even smell like a black hole. But it has one crucial difference: It has no event horizon, meaning that you can escape its gravitational clutches if you try hard enough.
It's called a Buchdahl star, and it is the densest object that can exist in the universe without becoming a black hole itself.
But no one has ever observed one, leading to questions about whether the mysterious objects actually exist. Now, a physicist may have uncovered a new property of Buchdahl stars that could help to answer that.
Black hole journeys
By and large, astronomers agree that black holes exist. We see evidence for them everywhere we look, including the release of gravitational waves when they collide and the dramatic shadows they carve out of surrounding materials. Astronomers also understand how black holes form: They are the remnants of the catastrophic gravitational collapse of massive stars. When giant stars die, no force in nature is capable of sustaining the stars' own weight, so these doomed behemoths just keep crushing themselves to infinity.
What astronomers currently don't understand, however, is how compressed an object can get without becoming a black hole. We know of white dwarfs, which contain a sun's worth of mass in a volume equivalent to Earth, and we know of neutron stars, which compress all that down even further into the volume of a city. But we don't know if there's anything smaller still that avoids the fate of becoming a black hole.
Buchdahl stars
In 1959, German-Australian physicist Hans Adolf Buchdahl explored how a highly idealized "star" — represented as a perfectly spherical blob of material — might behave as it was compressed as much as possible. As the blob got smaller and smaller, its density rose, making its own gravitational pull even more intense. Using the tools of Einstein's general theory of relativity, Buchdahl found an absolute lower limit to the size of that blob.
That special radius is equal to 9/4 times the mass of the blob, multiplied byNewton's gravitational constant, all divided by the speed of light squared.
Sign up for the Live Science daily newsletter now
Get the world’s most fascinating discoveries delivered straight to your inbox.
The Buchdahl limit is important because it defines the densest possible object that can still avoid becoming a black hole. Below that, the blob of material must always become a black hole, at least in the theory of relativity.
Living on the edge
Finding exotic objects that come right to the edge of that limit — so-called Buchdahl stars — has become a popular pastime of theorists and observationalists alike. Now, Naresh Dadhich, a physicist at the Inter-University Centre for Astronomy and Astrophysics in Pune, India, may have discovered a surprising property held by Buchdahl stars. Dadhich discusses this property in a new paper submitted Dec. 11 to the preprint server arXiv.org.
Dadhich, who calls Buchdahl stars "black hole mimics" because their observable properties would be nearly identical, studied what happens to the energy of a hypothetical star as it begins collapsing into a Buchdahl star.
"As the star collapses, it picks up gravitational potential energy, which is negative because gravity is attractive," Dadhich explained. At the same time, the interior of the star gains kinetic energy as all the particles are forced to jostle against each other in a smaller volume.
By the time the star reaches the Buchdahl limit, Dadhich found a surprising yet familiar relationship: The total kinetic energy was equal to half the potential energy.
This relationship is known as the virial theorem, and it applies to numerous situations in astronomy where the force of gravity is in balance with other forces. This means that a Buchdahl star could theoretically exist as a stable object with known, well-understood properties.
This finding suggests that theoretical Buchdahl stars may really be out there, and could lead to insights about the inner workings of black holes.
"There has always been attempts to define objects that are as close as possible to black holes," Dadhich said in an email to Live Science. "The event horizon of a black hole blocks our view of what's inside it. But we can interact with a Buchdahl star and study what it's made of, which may give us clues as to what black hole interiors are like."
Finding a Buchdahl star is another matter. To date, there is no known arrangement of matter that can create a Buchdahl star. But Dadhich's work points towards a path forward to understanding how they might work. Further research will be needed to discover what other properties these exotic objects might have, and what they might tell us about black holes.
Paul M. Sutter is a research professor in astrophysics at SUNY Stony Brook University and the Flatiron Institute in New York City. He regularly appears on TV and podcasts, including "Ask a Spaceman." He is the author of two books, "Your Place in the Universe" and "How to Die in Space," and is a regular contributor to Space.com, Live Science, and more. Paul received his PhD in Physics from the University of Illinois at Urbana-Champaign in 2011, and spent three years at the Paris Institute of Astrophysics, followed by a research fellowship in Trieste, Italy.